Confocal imaging
Laser scanning confocal microscopy is a significant advance in the field of optical microscopy, primarily because it permits sample visualization deeper within living and fixed cells, tissues and other samples.
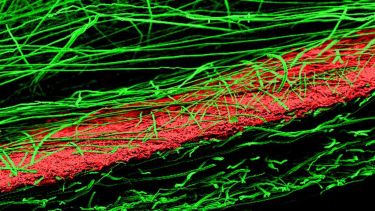
The confocal imaging facility is located in the Kroto building, North Campus, and is one of a number of analysis and characterisation facilities within the school.
The microscopes within this facility were supported by two BBSRC grants: BB/D524983/1 - Imaging of 3D Engineered Tissues and BB/E012981/1 - Two Photon Imaging: From Polymeric Materials to Engineered Tissues.
The facility contains two Zeiss LSM510 Meta confocal microscopes which can be booked and operated independently of each other. Both microscopes have their own set of lasers and both can also be connected to a tuneable two-photon Ti-Sapphire laser.
There is an offline PC, running the Zeiss image analysis software, which can be booked for data analysis.
There is also a Leica inverted epifluorescent microscope with an environmental chamber and camera, and an Olympus inverted epifluorescent microscope with camera.
This open access facility is available for use by researchers based in the Department of Materials Science and Engineering, other users from within Sheffield University and external researchers from both academia and industry.
The facility also supports users of the Zeiss Lightsheet z.1, and Cellvizio Dual Band Invivo confocal. These two pieces of equipment are within Royce@Sheffield, as part of the Henry Royce Institute
For further information or to discuss your requirements in more detail, please contact Dr Nicola Green.
- Widefield Fluorescence Microscopy
There is an inverted widefield fluorescent microscope in the Kroto Imaging area which is particularly good for imaging thin samples, even with low levels of fluorescence.
Available equipment
It is an IX73 Olympus microscope, with wLS LED illumination, Cairn MonoLED for brightfield imaging and a Retiga 6000 CCD camera. This microscope has 4x, 10x and 20x objectives and is capable of obtaining images from blue, green and red emitting fluorophores as well as standard brightfield images. The details of the excitation and emission filter set used can be found here, with the same filter cube being used at all times. The LEDs used to illuminate the sample are varied to generate images from the different fluorophores.
The 4x and 10x objectives can obtain images of cells grown on standard tissue culture well plates. The 20x objective requires a glass coverslip, or chamber optimised for optical imaging (e.g. Ibidi, Eppendorf, or Thermofisher chambered slides).
How to use the Olympus widefield microscopeFor advice in this area please contact Dr Nicola Green
- Confocal Imaging
Laser scanning confocal microscopy is a significant advance in the field of optical microscopy, primarily because it permits sample visualization deep within living and fixed cells, tissues and other samples. It provides the ability to collect sharply defined optical sections from which three-dimensional renderings can be created.
Please remember however, that the quality of the image that you will get from this process is greatly affected by the quality of your sample. Or to put it more simply - RUBBISH IN, RUBBISH OUT!!! Consequently, great care and planning is required for all stages of sample preparation to ensure a good image is obtained.
Why Use the Confocal Microscope
An epifluorescent microscope is capable of imaging some fluorescent samples very effectively. It uses an objective lens to focus light upon the specimen and to collect the light being emitted. However, any sample thicker than about 5 µm will appear indistinct and out of focus, because parts of the sample are outside the objective focal plane. In addition, epifluorescent microscopes generally use the line-spectrum produced by a high pressure mercury bulb for sample excitation, whereas a confocal microscope uses monochromatic lasers to excite the sample. This allows the user to choose very specific fluorescence excitation wavelengths and expands the range of fluorophores that can be used together on a single sample.
Advantages of confocal imaging
- Collects light from a single focal plane
- User can change the focal plane to obtain optical slices at increasing depths within sample. These can then be combined to produce 3D images
- No stray lateral interference, improving image contrast
- Specific wavelengths used to excite sample
Disadvantages of confocal imaging
- Signal strength reduced by requirement for detector pinhole
- Reduced signal to noise ratio so increased sensitivity to noise
- Technique is more labour intensive and requires more training and experience to be successful
Both confocal microscopes are connected to their own switchable Class 3b laser module which contains a number of lasers emitting light in the UV and visible spectral ranges. This provides each microscope with the following excitation capabilities
Argon/2 - 458, 477, 488, 514 nm
HeNe1 - 543 nm
HeNe2 - 633 nmBoth are also connected to a class 4 tuneable Ti-Sapphire two-photon laser capable of excitation wavelengths between 690 and 1040 nm.
Comparison of Single and Two-Photon Imaging
Advantages of Two-Photon Imaging
Optical sections can be obtained from deeper within the sample
- No attenuation of excitation source from outside focal plane
- Longer excitation wavelengths used which scatter less
- No pinhole required so all emitted light can be captured for imaging
Reduced damage to the sample
- No fluorophore excitation in bulk of specimen, production of phototoxins is therefore limited only to focal plane
- Excitation uses lower energy photons which have less impact on sample
Disadvantages of Two-Photon Imaging
- Slightly lower resolution
- Potential for thermal damage to specimen if it contains chromophores that absorb the excitation wavelengths
- Two-photon excitation spectra is not fully characterised for all fluorophores
In addition to standard imaging protocols the microscopes are able to perform non-descanned detector imaging (NDD), time-correlated single photon counting and second harmonic imaging.
Please contact Dr Nicola Green for more information.
- Including Confocal Images in Publications
Materials and Methods Information
The precise details of the imaging process are so variable between users and samples that it is difficult to provide a generic piece of text for inclusion in the Materials and Methods section of publications. However, below is a suggested template which you could use as a starting point, together with the addition of details specific to your own image acquisition process. Areas where you will need to replace the text with the specific details from your experiment are highlighted in bold to indicate this.
Images (pixel size x pixel size) were obtained using a Zeiss LSM 510Meta upright or inverted confocal microscope and objective details, with a pixel dwell time of dwell time.
Fluorophore 1 was excited using a laser wavelength nm laser (% transmission) and emission detected between [or "above" - if using a long pass filter] give wavelengths of light detected as a result of filter used.
All image analysis was performed using software for image analysis.
N.B. If more than one fluorophore was used to generate an image, it is necessary to give the details for each fluorophore separately.Acknowledging the Imaging Facility
If you include any images obtained from the Kroto Imaging Facility within your published work please ensure that you acknowledge the Kroto Imaging Facility.
The following is an example of the wording that you could use:-
Imaging work was performed at the Kroto Research Institute Confocal Imaging Facility, using the LSM510 Meta inverted/upright (delete as appropriate) confocal microscope.
And finally - if you also send Dr Nicola Green the details and, if possible, a PDF copy of any papers published as this documentation will help to justify the continued existence of the Facility, demonstrate its value to the research community and significantly enhance future funding applications.
- Henry Royce Equipment
The Kroto Confocal Facility is also home to the Lightsheet Z1 and Cellvizio confocal; two pieces of equipment from the Henry Royce Institute.
This equipment is available for use by industrial collaborators and academics within the Henry Royce Institute, as well as researchers here in Sheffield.
Please contact Dr Nicola Green or Royce@Sheffield.ac.uk if you require more information
- Recent Publications
The following are some of the publications which include images or data obtained using the Kroto Imaging Facility
Publication year
2023, 2022, 2021, 2020, 2019, 2018, 2017, 2016, 2015, 2014, 2013, 2012, 2011, 2010, 2009
Selectively inhibiting malignant melanoma migration and invasion in an engineered skin model using actin-targeting dinuclear Ru(II)-complexes. A. Raza, S.A. Archer, J.A. Thomas, S. MacNeil, J.W. Haycock. RSC Med Chem. 2023 14(1):65-73
Aligned Polyhydroxyalkanoate Blend Electrospun Fibers as Intraluminal Guidance Scaffolds for Peripheral Nerve Repair. C.S. Taylor, M. Behbehani, A Glen, P. Basnett, D.A. Gregory, B.B. Lukasiewicz, R. Nigmatullin, F. Claeyssens, I. Roy, J.W. Haycock ACS Biomater Sci Eng. 2023 epub ahead of print
Back to top of section
2022
Back to top of section
2021
In Vitro Low-Fluence Photodynamic Therapy Parameter Screening Using 3D Tumor Spheroids Shows that Fractionated Light Treatments Enhance Phototoxicity. J.R. Aguilar Cosme, D.C. Gagui, N.H. Green, H.E. Bryant, F. Claeyssens. ACS Biomater Sci Eng. 2021 7(11):5078-5089
Thiolene- and Polycaprolactone Methacrylate-Based Polymerized High Internal Phase Emulsion (PolyHIPE) Scaffolds for Tissue Engineering. B. Aldemir Dikici, A. Malayeri, C. Sherborne, S. Dikici, T. Paterson, L. Dew, P. Hatton, I. Ortega Asencio, S. MacNeil, C. Langford, N.R. Cameron, F. Claeyssens. Biomacromolecules (2021) doi: 10.1021/acs.biomac.1c01129
Harnessing Polyhydroxyalkanoates and Pressurized Gyration for Hard and Soft Tissue Engineering. P. Basnett, R.K. Matharu, C.S. Taylor, U. Illangakoon, J.I. Dawson, J.M. Kanczler, M. Behebhani, E. Humphrey, Q. Majid, B. Lukasiewicz, R. Nigmatullin, P. Heseltine, R.O.C. Oreffo, J.W. Haycock, C. Terracciano, S. Harding, M. Edirisinghe, I. Roy. ACS Appl Mater Interfaces (2021) 13(28):32624-32639
Electrospun Fiber Alignment Guides Osteogenesis and Matrix Organization Differentially in Two Different Osteogenic Cell Types. R.M. Delaine-Smith, A.J. Hann, N.H. Green, G.C. Reilly. Front Bioeng Biotechnol (2021) 25:672959.
Decellularised extracellular matrix decorated PCL PolyHIPE scaffolds for enhanced cellular activity, integration and angiogenesis. S. Dikici, B. Aldemir Dikici, S. MacNeil, F. Claeyssens. Biomater Sci (2021) 9(21):7297-7310.
A Dinuclear Osmium(II) Complex Near-Infrared Nanoscopy Probe for Nuclear DNA. F. Dröge, F. F. Noakes, S. A. Archer, S. Sreedharan, A. Raza, C. C. Robertson, S. MacNeil, J.W. Haycock, H. Carson, A.J.H.M. Meijer, C.G.W. Smythe, J. Bernadiona de la Serna. J. Am. Chem. Soc. 2021 143(48) 20442-20453
Bioresorbable and Mechanically Optimized Nerve Guidance Conduit Based on a Naturally Derived Medium Chain Length Polyhydroxyalkanoate and Poly(ε-Caprolactone) Blend. X. Mendibil, F. González-Pérez, X. Bazan, R. Díez-Ahedo, I. Quintana, F.J. Rodríguez, P. Basnett, R. Nigmatullin, B. Lukasiewicz, I. Roy, C.S Taylor, A. Glen, F. Claeyssens, J.W. Haycock, W. Schaafsma, E. González, B. Castro, P. Duffy, S. Merino. ACS Biomater Sci Eng (2021) 7(2):672-689
Fabrication of Topographically Controlled Electrospun Scaffolds to Mimic the Stem Cell Microenvironment in the Dermal-Epidermal Junction. D.H. Ramos-Rodriguez, S. MacNeil, F. Claeyssens, I. Ortega Asencio. ACS Biomater Sci Eng (2021) 7(6):2803-2813
Cell guidance on peptide micropatterned silk fibroin scaffolds. W. Sun, C.S. Taylor, Y. Zhang, D.A. Gregory, M.A. tomeh, J.W. Haycock, P.J. Smith, F. Wang, Q. Xia, X. Zhao. J Colloid Interf Sci (2021) 603:380-390
Cost effective optimised synthetic surface modification strategies for enhanced control of neuronal cell differentiation and supporting neuronal and Schwann cell viability. C.S. Taylor, R. Chen, R. D' Sa, J.A. Hunt, J.M. Curran, J.W. Haycock. J Biomed Mater Res B Appl Biomater (2021) 109(11):1713-1723
Back to top of section
2020
Design and Evaluation of an Osteogenesis-on-a-Chip Microfluidic Device Incorporating 3D Cell Culture. H. Bahmaee, R. Owen, L. Boyle, C.M. Perrault, A.A. Garcia-Granada, G.C. Reilly, F. Claeyssens. Front Bioeng Biotechnol (2020) 8:557111
Assessment of the Angiogenic Potential of 2-Deoxy-D-Ribose Using a Novel in vitro 3D Dynamic Model in Comparison With Established in vitro Assays. S. Dikici, B. Aldemir Dikici, S.I. Bhaloo, M. Balcells, E.R. Edelman, S. MacNeil, G.C. Reilly, C. Sherborne, F. Claeyssens. Front Bioeng Biotechnol (2020) 7:451
2-deoxy-d-ribose (2dDR) upregulates vascular endothelial growth factor (VEGF) and stimulates angiogenesis. S. Dikici, A.J. Bullock, M. Yar, F. Claeyssens, S. MacNeil. Microvasc Res (2020) 131:104035
Pre-Seeding of Simple Electrospun Scaffolds with a Combination of Endothelial Cells and Fibroblasts Strongly Promotes Angiogenesis. S. Dikici, F. Claeyssens, S. MacNeil. Tissue Eng Regen Med (2020) 17(4):445-458
Bioengineering Vascular Networks to Study Angiogenesis and Vascularization of Physiologically Relevant Tissue Models in Vitro. S. Dikici, F. Claeyssens, S. MacNeil. ACS Biomater Sci Eng (2020) 6(6):3513-3528
UV-Casting on Methacrylated PCL for the Production of a Peripheral Nerve Implant Containing an Array of Porous Aligned Microchannels. R. Diez-Ahedo, X. Mendibil, M.C. Márquez-Posadas, I. Quintana, F. González, F.J. Rodríguez, L. Zilic, C. Sherborne, A. Glen, C.S Taylor, F. Claeyssens, J.W. Haycock, W. Schaafsma, E. González, B. Castro, S. Merino. Polymers (2020) 12(4):971
Modulation of neuronal cell affinity of composite scaffolds based on polyhydroxyalkanoates and bioactive glasses. L.R. Lizarraga-Valderrama, R. Nigmatullin, B. Ladino, C.S. taylor, A.R. Boccaccini, J.C. Knowles, F. Claeyssens, J.W. Haycock, I. Roy. Biomed Mat (2020) 15:045024
Combined Porogen Leaching and Emulsion Templating to produce Bone Tissue Engineering Scaffolds. R. Owen, C. Sherborne, R. Evans, G.C Reilly, F. Claeyssens. Int J Bioprint (2020) 6(2):265
A Dinuclear Ruthenium(II) Complex Excited by Near-Infrared Light through Two-Photon Absorption Induces Phototoxicity Deep within Hypoxic Regions of Melanoma Cancer Spheroids. A. Raza, S.A. Archer, S.D. Fairbanks, K.L. Smitten, S.W. Botchway, J.A. Thomas, S. MacNeil, J.W. Haycock. J Am Chem Soc (2020) 142:4639-4647
Biomimetic surface delivery of NGF and BDNF to enhance neurite outgrowth. A.M. Sandoval-Castellanos, F. Claeyssens, J.W. Haycock. Biotech Bioeng (2020) 1-12
Back to top of section
2019
Carbon dot-protoporphyrin IX conjugates for improved drug delivery and bioimaging. J.R. Aguilar Cosme, H.E. Bryant, F. Claeyssens. PLOS ONE (2019) 14(7): e0220210
A Novel Bilayer Polycaprolactone Membrane for Guided Bone Regeneration: Combining Electrospinning and Emulsion Templating. B. Aldemir Dikici, S. Dikici, G.C. Reilly, S. MacNeil, F. Claeyssens. Materials (2019) 12(16):2643
A dinuclear ruthenium(II) phototherapeutic that targets duplex and quadruplex DNA. S. Archer, A. Raza, F, Drohe, C. Robinson. A.J. Auty, D. Chekulaev, J.A. Weinstein, T. Keane, A.J.H.M. Meijer, J.W. Haycock, S. MacNeil, J.A. Thomas. Chem Sci (2019) 10:3502-3513
Slow polymer diffusion on brush-patterned surfaces in aqueous solution. C.G. Clarkson, A. Johnson. G.J. Leggett, M. Geoghegan. Nanoscale (2019) 11:6052-6061
Unidirectional neuronal cell growth and differentiation on aligned polyhydroxyalkanoate blend microfibres with varying diameters. L.R. Lizarraga-Valderrama, C.S. Taylor, F. Claeyssens, J.W. Haycock, J. Knowles, I. Roy. J Tissue Eng Regen Med (2019) 13:1581-1594
Two photon excitable graphene quantum dots for structured illumination microscopy and imaging applications: lysosome specificity and tissue-dependent imaging. H. Singh, S. Sreedharan, K. Tiwari, N.H. Green, C. Smythe, S.K. Pramanik, J.A. Thomas, A. Das. Chem Comm (2019) 55:521-524
Back to top of section
2018
Pre-clinical evaluation of advanced nerve guide conduits using a novel 3D in vitro testing model. M. Behbehani, A. Glen, C.S. Taylor, A. Schuhmacher, F. Claeyssens, J.W. Haycock, Int J Bioprint (2018) 4(1):UNSP 123
Composite porous scaffold of PEG/PLA support improved bone matrix deposition in vitro compared to PLA-only scaffolds. B. Bhaskar, R. Owen, H. Bahmaee, Z. Wally, P. Sreenivasa Rao, G.C. Reilly, J Biomed Mat Res (2018) 6(5):1334-1340
An Improved In Vivo Methodology to Visualise Tumour Induced Changes in Vasculature Using the Chick Chorionic Allantoic Membrane Assay. N. Mangir, A. Raza, J.W. Haycock, C. Chapple, S. MacNeil, In Vivo (2018) 32(3):461-472
Synthesis, Characterization and 3D Micro-Structuring via 2-Photon Polymerization of Poly(glycerol sebacate)-Methacrylate-An Elastomeric Degradable Polymer. S. Pashneh-Tala, R. Owen, H. Bahmaee, S. Rekstyte, M. Malinauskas, F. Claeyssens Front Phys (2018) 6:41
Porous microspheres support mesenchymal progenitor cell ingrowth and stimulate angiogenesis. T.E. Paterson, G. Gigliobianco, C. Sherborne, N.H. Green, J.M. Dugan, S. MacNeil, G.C Reilly, F. Claeyssens, APL Bioengineering (2018) 2:026103
Additive manufactured biodegradable poly(glycerol sebacate methacrylate) nerve guidance conduits. D. Singh, A.J. harding, E. Albadawi, F.M. Boissonade, J.W. Haycock, F. Claeyssens. Acta Biomateriala (2018) 78:48-63
Back to top of section
2017
Measurement of friction-induced changes in pig aorta fibre organization by non-invasive imaging as a model for detecting the tissue response to endovascular catheters. L. Bostan, C. Noble, N. Smulders, R. Lewis, M.J. Carre, S.E. Franklin, N.H. Green, S. MacNeil, Biotribology (2017) 12:24
Poly(n-butyl Methacrylate) with Primary Amine End Groups for Supporting Cell Adhesion and Proliferation of Renal Epithelial Cells. K. Cox-Nowak, O. Al-Yamani, C.A. Grant, N.H. Green, S. Rimmer, Int J Polymeric Mat Polymetic Biomat (2017) 66(15):762-767
Development of a UV crosslinked biodegradable hydrogel containing adipose derived stem cells to promote vascularization for skin wounds and tissue engineering. G. Eke, N. Mangir, N. Hasirci, S. MacNeil, V. Hasirci, Biomaterials (2017) 129:188-198
A new mode of contrast in biological second harmonic generation microscopy. N.H. Green, R. Delaine-Smith, H.J. Askew, R. Byers, G.C. Reilly, S.J. Matcher, Sci Rep (2017) 7:13331
Selective laser-melting enabled electrospinning: Introducing complexity within electrospun membranes. T.E. Paterspn, S.N. Beal, M.E. Santocildes-Romero, A.T. Sidambe, P.V. Hatton, I.O. Ascencio. Proc Insti Mech Eng Part H - J Eng in Med (2017) 231:565-574
Imaging cellular trafficking processes in real time using lysosome targeted up-conversion nanoparticles. S.K. Pramanik, S. Sreedharan, H. Singh, N.H. Green, C. Smythe, J. Thomas, A. Das, Chem Com (2017) 53:12672
Oxygen Mapping of Melanoma Spheroids using Small Molecule Platinum Probe and Phosphorescence Lifetime Imaging Microscopy. A. Raza, H.E. Colley, E. Baggaley, I.V. Sazanovich, N.H. Green, J.A. Weinstein, S.W. Botchway, S. MacNeil, J.W. Haycock, Sci Rep (2017) 7:10743
Back to top of section
2016
Investigating Neovascularization in Rat Decellularized Intestine: An In Vitro Platform for Studying Angiogenesis. L. Dew, W.R. English, C.K. Chong, S. MacNeil, Tissue Eng Part A (2016) 22(23-24):1317-1326
Fabrication of Biodegradable Synthetic Vascular Networks and Their Use as a Model of Angiogenesis. L. Dew, W.R. English, I. Ortega, F. Claeyssens, S. MacNeil, Cells Tissues Organs (2016) 202(5-6):319-328
Antimicrobial Graft Copolymer Gels. A.C. Harvey, J. Madsen, C.W.I Douglas, S. MacNeil, S.P. Armes, Biomacromolecules (2016) 17(8):2710-2718
Developing Repair Materials for Stress Urinary Incontinence to Withstand Dynamic Distension. C.J. Hillary, S. Roman, A.J. Bullock, N.H. Green, C.R. Chapple, S. MacNeil, PLoS ONE (2016) 11(3):e0149971
Photochemically modified diamond-like carbon surfaces for neural interfaces A.P. Hopper, J.M. Dugan, A.A. Gill, E.M. Regan, J.W. Haycock, S. Kelly, P.W. May, F. Claeyssens, Mat Sci Eng C (2016) 58(1):1199–1206
Second Harmonic Generation microscopy reveals collagen fibres are more organised in the cervix of postmenopausal women. B.F. Narice, N.H. Green, S. MacNeil, D. Anumba, Reprod Biol Endocrinol (2016) 14(1):70
Controlled peel testing of a model tissue for diseased aorta. C. Noble, N. Smulders, R. Lewis, M.J. Carre, S.E Franklin, S. MacNeil, Z.A. Taylor, J Biomech (2016) 49(15):3667-3675
Creating a model of diseased artery damage and failure from healthy porcine aorta. C. Noble, N. Smulders, N.H. Green, R. Lewis, M.J. Carre, S.E. Franklin, S. MacNeil, Z.A. Taylor, J Mech Behav Biomed Mat (2016) 60:378-393
Production and characterization of a novel, electrospun, tri-layer polycaprolactone membrane for the segregated co-culture of bone and tissue. S. Puwanun, F.J. Bye, M.M. Ireland, S. MacNeil, G.C. Reilly, N.H. Green, Polymers (2016) 8(6):221-229
Combining 3D human in vitro methods for a 3Rs evaluation of novel titanium surfaces in orthopaedic applications. G. Stevenson, S. Rehman, E. Draper, E, Hernandex-Nava, J. Hunt, J.W. Haycock, Biotech Bioeng (2016) 113(7):1586-1599
Inkjet printing Schwann cells and neuronal analogue NG108-15 cells. C. Tse, R. Whitely, T. Yu, J. Stringer, S. MacNeil, J.W. Haycock, P.J. Smith, Biofabrication (2016) 8(1):015017
Utilising Inkjet Printed Paraffin Wax for Cell Patterning Applications C.C.W. Tse, S.S. Ng, J. Stringer, S. MacNeil, J.W. Haycock, P.J. Smith, Int J Bioprint (2016) 2(1) doi:10.18063/ijb.2016.01.001
Decellularisation and histological characterisation of porcine peripheral nerves. L. Zilic, S.P. Wilshaw, J.W. Haycock, Biotech Bioeng (2016) 113(9):2041-2053
Back to top of section
2015
Characterization of diblock copolymer order-order transitions in semi-dilute aqueous solution using fluorescence correlation spectroscopy C.G. Clarkson, J.R. Lovett, J. Madsen, S.P. Armes, M. Geoghegan, Macromol Rapid Commun (2015) 36(17):1572-1577
Rocking media over ex vivo corneas improves this model and allows the study of the effect of proinflammatory cytokines on wound healing. P. Deshpande, Í. Ortega, F. Sefat, V.S. Sangwan, N. Green, F. Claeyssens, S. MacNeil, Invest Ophthalmol Vis Sci (2015) 56(3):1553-1561
Thermoresponsive, stretchable, biodegradable and biocompatible poly(glycerol sebacate)-based polyurethane hydrogels. M. Frydrych, S. Román, N.H. Green, S. MacNeil, B. Chen Polym Chem (2015) 6:7974-7987
Simple surface coating of electrospun poly-L-lactic acid scaffolds to induce angiogenesis. G. Gigliobianco, C.K. Chong, S. MacNeil, J Biomater Appl (2015) 30:50-60
Towards the fabrication of artificial 3D microdevices for neural cell networks. A.A. Gill, Í Ortega, S. Kelly, F. Claeyssens, (2015) 17(2):27
Nerve tissue engineering using blends of poly(3-hydroxyalkanoates) for peripheral nerve regeneration. L.R. Lizarraga-Valderrama, R. Nigmatulin, C. Taylor, J.W. Haycock, F. Claeyssens, J.C. Knowles, I Roy Eng Life Sci (2015) 15(6):612-621
Fabrication of biodegradable synthetic perfusable vascular networks via a combination of electrospinning and robocasting. Í. Ortega, L. Dew, A.G. Kelly, C.K. Chong, S. MacNeil, F. Claeyssens, Biomater Sci. (2015) 3(4):592-596
Emulsion templated scaffolds with tunable mechanical properties for bone tissue engineering. R. Owen, C. Sherborne, T. Paterson, N.H. Green, G.C. Reilly, F. Claeyssens, J Mech Behav Biomed Mater (2015) 54:159-172
Nerve guides manufactured from photocurable polymers to aid peripheral nerve repair. C.J. Pateman, A.J. Harding, A. Glen, C.S. Taylor, C.R. Christmas, P.P. Robinson, S. Rimmer, F.M. Boissonade, F. Claeyssens, J.W. Haycock, Biomaterials (2015) 49:77-89
Arginine–glycine–aspartic acid functional branched semi-interpenetrating hydrogels. R.A. Plenderleith, C.J. Pateman, C. Rodenburg, J.W. Haycock, F. Claeyssens, C. Sammon, S. Rimmer, Soft Matter (2015) 11(38):7567-78
An anatomical study of porcine peripheral nerve and its potential use in nerve tissue engineering. L. Zilic, P.E. Garner, T. Yu, S. Román, J.W. Haycock, S.-P. Wilshaw, J Anat (2015) 227(3):302-314
Back to top of section
2014
Dinuclear Ruthenium(II) Complexes as Two-Photon, Time-Resolved Emission Microscopy Probes for Cellular DNA. E. Baggaley, M.R. Gill, N.H. Green, D. Turton, I.V. Sazanovich, S.W. Botchway, C. Smythe, J.W. Haycock, J.A. Weinstein, J.A. Thomas, Angew Chem Int Ed Engl (2014) 53(13):3367-3371
Two-photon phosphorescence lifetime imaging of cells and tissues using a long-lived cyclometallated Npyridyl^Cphenyl^Npyridyl Pt(II) complex. E. Baggaley, I.V. Sazanovich, J.A.G. Williams, J.W. Haycock, S.W. Botchway, J. Weinstein, RSC Advances (2014) 4:35003-35008
Monitoring Fibrous Scaffold Guidance of Three-Dimensional Collagen Organisation Using Minimally-Invasive Second Harmonic Generation. R. Delaine-Smith, N.H. Green, S.J. Matcher, S. MacNeil, G.C. Reilly, PLOS ONE (2014) 9(2):0089761
Primary cilia respond to fluid shear stress and mediate flow-induced calcium deposition in osteoblasts. R.M. Delaine-Smith, A. Sittichokechaiwut, G.C. Reilly, FASEB J (2014) 28(1):430-439
Amine functional hydrogels as selective substrates for corneal epithelialization. E. Hassan, P. Deshpande, F. Claeyssens, S. Rimmer, S. MacNeil, Acta Biomater (2014) 10(7):3029-3037
Amine functionalized nanodiamond promotes cellular adhesion, proliferation and neurite outgrowth. A.P. Hopper, J.M. Dugan, A.A. Gill, O.J.L. Fox, P.W. May, J.W. Haycock, F. Claeyssens, Biomed Mater (2014) 9(4):045009
A mechanistic dissection of polyethylenimine mediated transfection of CHO cells: to enhance the efficiency of recombinant DNA utilization. O.L. Mozely, B.C. Thompson, A. Fernandez-Martell, D.C. James, Biotechnol Prog (2014) 30(5):1161-1170
Combination of microsterolithography and electrospinning to produce membranes equipped with niches for conreal regeneration. I. Ortega, F. Sefat, P. Deshpande, T. Paterson, C. Ramachandran, A.J. Ryan, S MacNeil, F. Claeyssens, J Vis Exp (2014) 12(91):51826
Development of a one-step approach for the reconstruction of full thickness skin defects using minced split thickness skin grafts and biodegradable synthetic scaffolds as a dermal substitute. K. Sharma, A. Bullock, D. Ralston, S. MacNeil, Burns (2014) 40(5):957-965
Back to top of section
2013
The development of a 3D immunocompetent model of human skin. D.Y. Chau, C. Johnson, S. MacNeil, J.W. Haycock, A.M. Ghaemmaqhami, Biofabrication (2013) 5(3):035011
Simplifying corneal surface regeneration using a biodegradable synthetic membrane and limbal tissue explants. P. Deshpande, C. Ramachandran, F. Sefat, I. Mariappan, C. Johnson, R. McKean, M. Hannah, V.S. Sangwan, F. Claeyssens, A.J. Ryan, S. MacNeil, Biomaterials (2013) 34(21):5088-5106
Generic methods for micrometer- and nanometer-scale surface derivatization based on photochemical coupling of primary amines to monolayers of aryl azides on gold and aluminum oxide surfaces O. El Zubir, I. Barlow, E. Ul-Haq, H.A. Tajuddin, N.H. Williams, G.J. Legget, Langmuir (2013) 29(4):1083-1092
Development of a microfabricated artifical limbus with micropockets for cell delivery to the cornea. I. Ortega, P. Deshpande, A.A. Gill, S. MacNeil, F. Claeyssens, Biofabrication (2013) 5(2):025008
Combined microfabrication and electrospinning to produce 3-D architectures for corneal repair. I. Ortega, A.J. Tyan, P. Deshpande, S. MacNeil, F. Claeyssens, Acta Biomaterialia (2013) 9(3):5511-5520
Laser exposure of gold nanorods can induce intracellular calcium transients. C. Paviolo, J.W. Haycock, P.J. Cadusch, S.L. McArthur, P.R. Stoddart, J Biophotonics (2013) 7(10):761-765
Laser exposure of gold nanorods can increase neuronal cell outgrowth. C. Paviolo, J.W. Haycock, J. Yong, A. Yu, P.R. Stoddart, S.L. McArthur, Biotech Bioeng (2013) 110(8):2277-2291
Back to top of section
2012
The diffusion of dextran within poly(methacrylic acid) hydrogels. A.M. AL-Baradi, M. Mears, R.A.L. Jones, M. Geoghegan, J Polym Sci B: Polym Phys (2012) 50:1286-1292
An aligned 3D neuronal-glial co-culture model for peripheral nerve studies. M.F.B. Daud, K.C. Pawar, F. Claeyssenss, A.J. Ryan, J.W. Haycock, Biomaterials (2012) 33(25):5901-5913
Matrix production and collagen structure are enhanced in two types of osteogenic progenitor cells by a simple fluid shear stress stimulus. R.M. Delaine-Smith S. MacNeil, G.C. Reilly, Eur Cell Mater (2012) 24:162-174
Integrated culture and purification of rat Schwann cells from freshly isolated adult tissue R. Kaewkhaw, A.M. Scutt, J.W. Haycock, Nature Protocols (2012) 7:1996-2004
Two-photon polymerization-generated and micromolding-replicated 3D scaffolds for peripheral neural tissue engineering applications A. Koroleva, A.A. Gill, I. Ortega, J.W. Haycock, S. Schlie, S.D. Gittard, B.N. Chichkov, F. Claeyssens, Biofabrication (2012) 4:025005
Micrometer and Nanometer Scale Photopatterning of Proteins on Glass Surfaces by Photo-degradation of Films Formed from Oligo(Ethylene Glycol) Terminated Silanes G. Tizazu, O. El Zubir, S. Patole, A. McLaren, C. Vasilev, D.J. Mothersole, A. Adawi, D.G. Lidzey, G.P. Lopez, G.J. Legget, Biointerphases (2012) 7(1-4):54-63
Back to top of section
2011
Protein Micro- and Nanopatterning Using Aminosilanes with Protein-Resistant Photolabile Protecting Groups. S.A. Alang Ahmad, L.S. Wong, E. ul-Haq, J.K. Hobbs, G.J. Leggett, J. Micklefield, J Am. Chem. Soc. (2011) 133(8):2749-2759
Magnetic field dependence of the diffusion of single dextran molecules within a hydrogel containing magnetite nanoparticles. A.M. Al-Baradi, O.O. Mykhaylyk, H.J. Blythe, M. Geoghegan, J Chem Phys (2011) 134:094901
Biocompatible hydrogels based on hyaluronic acid cross-linked with a polyaspartamide derivative as delivery systems for epithelial limbal cells. C. Fiorica, R.A. Senior, G. Pitarresi, F.S. Palumbo, G. Giammona, P. Deshpande, S. MacNeil, Int J Pharm (2011) 414(1-2):104-111
Ruthenium(II) metallo-intercalators: DNA imaging and cytotoxicity. M.R. Gill, H. Derrat, C.G. Smythe, G. Battaglia, J.A. Thomas, Chembiochem (2011) 12(6):887-880
NF-kB is activated in oesophageal fibroblasts in response to a paracrine signal generated by acid-exposed primary oesophageal squamous cells. N.H. Green, Q. Huang, B.M. Corfe, J.P. Bury, S. MacNeil, Int J Exp Pathol (2011) 92(5):345-356
Tracking nanoparticles in three-dimensional tissue-engineered models using confocal laser scanning microscopy. V. Hearnden, S. MacNeil, G. Battaglia, Methods Mol Biol (2011) 695:41-51
Anatomical site influences the differentiation of adipose-derived stem cells for Schwann-cell phenotype and function. R. Kaewkhaw, R.M. Scutt, J.W. Haycock, Glia (2011) 59(5):734-749
Direct laser writing of 3D scaffolds for neural tissue engineering applications. V. Melissinaki, A.A. Gill, I. Ortega , M. Vamvakaki, A. Ranella A, J.W. Haycock, C. Fotakis, M. Farsari, F. Claeyssens, Biofabrication (2011) 3(4):045005
Three-dimensional alignment of schwann cells using hydrolysable microfiber scaffolds: strategies for peripheral nerve repair. C. Murray-Dunning, S.L. McArthur, T. Sun, R. McKean, A.J. Ryan, J.W. Haycock, Methods Mol Biol (2011) 695:155-166
Degradation, Bioactivity, and Osteogenic Potential of Composites Made of PLGA and Two Different Sol–Gel Bioactive Glasses. E. Pamula, J. Kokoszka,K. Cholewa-Kowalska, M. Laczka, L. Kantor, L. Niedzwiedzki, G.C. Reilly, J. Filipowska, W. Madej, M. Kolodziejczyk, G. Tylko, A.M. Osyczka, Ann Biomed Eng (2011) 39(8):2114-2129
Back to top of section
2010
Protein Patterning by UV-Induced Photodegradation of Poly(oligo(ethylene glycol) methacrylate) Brushes. S.A. Alang Ahmad, A. Hucknall, A. Chilkoti, G.J. Leggett, Langmuir (2010) 26(12):9937-9972
Biofilm formation in environmental bacteria is influenced by different macromolecules depending on genus and species. J.S. Andrews, S.A. Rolfe, W.E. Huang, J.D. Scholes, S.A. Banwart, Environ Microbiol (2010) 12(9):2496-2507
Using poly(lactide-co-glycolide) electrospun scaffolds to deliver cultured epithelial cells to the cornea. P. Deshpande, R. McKean, K.A. Blackwood, R.A. Senior, A. Ogunbanjo, A.J. Ryan, S. MacNeil, Regen. Med. (2010) 5(3):395-401
Enhanced fluorescence imaging of live cells by effective cytosolic delivery of probes. M. Massignani, I. Canton, T. Sun, V. Hearnden, S. MacNeil, A. Blanazs, S.P. Armes, A. Lewis, G. Battaglia, PLoS One (2010) 5(5):e10459
A micro-incubator for cell and tissue imaging. C. Picard, V. Hearnden, M. Massignani, S. Achouri, G. Battaglia, S. MacNeil, A. Donald, Biotechniques (2010) 48:135-138
Mechanisms of fluid-flow-induced matrix production in bone tissue engineering. H.L. Morris, C.I. Reed, J.W. Haycock, G.C. Reilly, Proc Inst Mech Eng H (2010) 224(12):1509-21
Intrinsic extracellular matrix properties regulate stem cell differentiation. G.C. Reilly, A.J. Engler, J Biomech (2010) 43(1):55-62
Short bouts of mechanical loading are as effective as dexamethasone at inducing matrix production by human bone marrow mesenchymal stem cells. A. Sittichokechaiwut, J.H. Edwards, A.M. Scutt, G.C. Reilly, Eur Cell Mater (2010) 20:45-57
Back to top of section
2009
Directed single molecule diffusion triggered by surface energy gradients. P. Burgos, Z. Zhang, R. Golestanian, G.J. Leggett, M. Geoghegan, ACS Nano (2009) 3:3235-43
A ruthenium(II) polypyridyl complex for direct imaging of DNA structure in living cells. M.R. Gill, J Barcia-Lara, S.J. Foster, C. Smythe, G. Battaglia, J.A. Thomas, Nat Chem (2009) 1:662-667
Diffusion Studies of Nanometer Polymersomes Across Tissue Engineered Human Oral Mucosa. V. Hearnden, H. Lomas, S. MacNeil, M. Thornhill, C. Murdoch, A. Lewis, J. Madsen, A. Blanazs, S. Armes, G. Battaglia, Pharmaceutical Research (2009) 26:1718-1728
Time-resolved and two-photon emission imaging microscopy of live cells with inert platinum complexes. S.W. Botchway, M. Charnley, J.W. Haycock, A.W. Parker, D.L. Rochester, J.A. Weinstein, J.A. Williams, Proc Natl Acad Sci USA (2008) 105(42):16071-6
Back to top of section
If you have published any data/images acquired using the Kroto Imaging Facility, please send the details to Dr Nicola Green, so it can be included in this list
- Registering as a New User
If you wish to access any of the equipment as a new user please contact Dr Nicola Green or Vanessa Singleton to discuss your requirements