Reconstructing a nuclear meltdown in Sheffield
Going inside a melted down nuclear reactor is a dangerous activity. But without knowing the environment inside, it’s impossible to decommission it safely. Dr Claire Corkhill and her team are attempting to recreate Chernobyl’s meltdown in Sheffield, in a bid to safely deactivate both power plants.
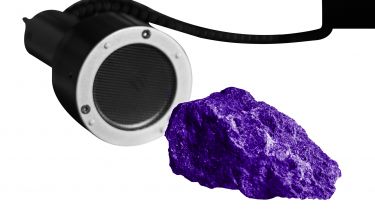
Decommissioning a nuclear reactor
Decommissioning a nuclear reactor means safely removing all the radioactive fuel and contaminated infrastructure and deconstructing the building piece by piece. Normally this happens when the reactor has reached the end of its working life, as is the case with Magnox reactors at Sellafield on the north-west coast of the UK. But Chernobyl’s end was very much unplanned. Dr Claire Corkhill is a Research Fellow in the Department of Material Science and Engineering at the University of Sheffield. She and her team have been working with Ukrainian scientists to understand what’s going on inside the extraordinary environment of Chernobyl's stricken reactor unit 4—and how it can be decommissioned safely. However, that also means navigating the challenges of a country with the second lowest GDP per capita in Europe.
“It costs a lot of money to tackle a project of this magnitude and in Ukraine they don’t have quite enough.” Claire explains. “The European Bank for Reconstruction and Development are bank-rolling the decommissioning operation, but it’s still down to scientists to understand how to safely remove the melted fuel.”
“They have guys who go into the reactor to assess the status of the fuel, which is corroding badly and creating radioactive dust. It’s someone’s job and they get a high dose of radiation doing it,” says Claire. Entering the reactor to see the extent of the chaos inside means risking high doses of radiation, but when robotic tools aren’t available, this is the only option.
The last major fuel sampling campaign was made in 1991, when a team of researchers, including a scientist named Dr Boris Burakov, from the Khlopin Radium Institute in St Petersburg, entered the building and made a beeline for the melted fuel to collect a sample.
But how did the inside of the reactor come to be such a chaotic space? “At the time of the disaster, the reactor would have reached temperatures higher than 2000 degrees celsius,” explains Claire. The melted core flowed down into the reactor building in a way that resembled lava rolling down the side of a volcano, melting concrete and stainless steel reactor components as it went. When the flow finally began to slow down and come to a halt it created an unusual shape which became known as the ‘elephants’ foot’.
This lethal radioactive structure, containing fuel from the reactor, quite literally became a target for the Russian scientists. Reportedly using a gun—because it was too hard to break with other tools — they shot at the seemingly impenetrable mass to break a bit off so it could be studied to understand exactly what it was and how it was formed. These pieces of fuel debris, the only samples of radioactive Chernobyl 'lava' in the world, would become a fundamental resource for Claire and her team 17 years later.
Knowing the situation inside the reactor is necessary to decommission it. But sending someone inside the reactor isn’t always an option, and the high doses of radiation and risks of roof collapse certainly don't make it a safe one. Without sending people inside how do we know what’s happening? This is the problem being faced over 5000 miles away at the Fukushima Daiichi Nuclear power plant.
The key to a solution
On the 11 March 2011, a 15 metre tsunami breached the protective walls surrounding the Fukushima Daiichi power plant, cutting off the power supply and cooling system for the reactors. In the first 72 hours all three reactor cores largely melted, creating an environment similar to the Chernobyl reactor — full of glassy radioactive material and elephant's feet — with the fuel trapped inside the reactor. “Until we remove the nuclear fuel there will always be a risk of radioactive material from the reactors reaching the environment and impacting upon current and future populations,” explains Claire. “The nuclear reactor materials will be radioactive and potentially harmful to people for 100,000 years.”
To remove the fuel from the reactor we need to fully understand the situation inside, which includes the mechanical properties of the ‘nuclear fuel debris’, a combination of radioactive building material, waste fuel and anything else that got in the way. But this is particularly difficult at Fukushima because, unlike Chernobyl, nobody has entered the reactor core to see the internal mess of the meltdown. And right now, there’s no plans for anyone to see it.
Sending people inside the reactor to assess the damage is a no go for the Japanese government. The threat is too great. However, the lack of access to the plant’s core means it’s difficult to know the mechanical properties of the fuel which is necessary to safely decommissioning it. But thanks to the team of Russian researchers who went into Chernobyl in 1991 Claire holds a key to unlocking the mystery inside the Fukushima reactor: the melted fuel sample.
Reconstructing a nuclear meltdown
Heading inside the reactor is treacherous, with threats around every corner, from the radioactivity itself to the instability of the building. Thankfully, Claire and her team prevent the need for anyone else to enter either destroyed reactor. The team are reconstructing what the fuel inside both the Fukushima and Chernobyl reactors might look like — both physically and chemically — using the melted fuel sample taken from Chernobyl as a reference. “What we’ve been doing is trying to recreate what the fuel looks like in Chernobyl in our labs in Sheffield. If we can accurately recreate it then we can use the same process to create a Fukushima fuel simulant, which is essential to help prepare for decommissioning operations and the removal of the fuel,” explains Claire.
As the fuel is corroding in each reactor it’s producing tonnes of tiny particles of radioactive dust. If it escapes into the air it will contaminate the workers trying to decommission the reactors, and may even enter the local environment. Understanding how the fuel corrodes means we can estimate how much radioactive dust there might be and how to mitigate the problem. “At Chernobyl over 30 tonnes of radioactive dust has been generated from the melted down nuclear fuel and this presents a serious potential environmental contamination risk,” says Claire.
As well as an awareness of how the fuel corrodes, the team need to know how hard the debris itself is so engineers can design robots that are capable of cutting it. Without this information the fuel will remain trapped in the reactor core. Although, before any of this can happen the team need to actually recreate the fuel, but how do you create conditions in a lab suitable for producing the chaotic core of a melted reactor? It turns out it’s a bit like baking a cake.
“We’re taking all of the ingredients from the Fukushima reactor — uranium dioxide fuel, zirconium fuel cladding, stainless steel and concrete — and melting them together at temperatures close to those from the real accident,” Claire says. When reconstructing the melted nuclear fuel, Claire and her team have left out the highly radioactive by-products formed in the fuel during the nuclear fission reaction that generates electricity. This makes it much less radioactive, meaning corrosion and mechanical testing experiments can be performed easily using state-of-the-art equipment at the University of Sheffield — without the need for shielding from high levels of radioactivity.
The end result is a component not too dissimilar from the sample taken from Chernobyl which can be tested by Claire and her team. One of the key findings so far, is that the fuel debris corrodes more like glass than nuclear fuel, which means that the tiny radioactive particles formed in the corrosion reaction are quite sticky and will not be released easily into the air.
Whilst this might help the Japanese and Russian governments with the problem of how to remove the fuel efficiently and without expelling large amounts of radioactive dust, it doesn’t quite solve a problem presented by the plutonium itself. Plutonium, the element with the highest atomic number in nature, is one of the earth’s most unstable elements. The Fukushima reactors used a mixture of plutonium dioxide and uranium dioxide as fuel (known as mixed oxide fuel, or MOX), which means there is plenty of it within the melted nuclear fuel.
The plutonium problem
“When too much plutonium is put together, there's a risk it will ‘go critical’,” explains Claire. In a nuclear reactor the fuel — uranium — undergoes a controlled nuclear fission reaction. That’s how nuclear energy produces electricity. A criticality event is an uncontrolled nuclear fission reaction. “It was criticality that made the Hiroshima bomb happen, and it works the same for plutonium as it does with uranium. Put too much together and you get a huge explosion and massive release of radioactivity,” says Claire.
The Japanese government is concerned that there’s still high amounts of plutonium in the fuel inside the reactors. “When removing the fuel and putting it into containers, there’s a risk of putting too much plutonium together because you don’t know how it’s distributed in the fuel debris. This can then go critical and cause an explosion,” says Claire. But there’s also a risk if the fuel remains inside the reactor because, again, it’s uncertain how much plutonium is in there. In theory it could go critical at any moment.
Understanding the distribution of plutonium inside the reactor is therefore fundamental to safely sorting the fuel as it’s removed from the reactor. To do this, Claire has added a non-radioactive alternative to plutonium into the reconstruction of nuclear fuel debris. The surrogate known as cerium has similar chemical properties to plutonium meaning it behaves in a nearly identical way in the mixture.
To see down to the level of cerium atoms and look at how they distribute in the debris, the team travelled over the Atlantic to the USA to use the world’s brightest microscopes. Here, they were able to see how cerium distributed in the mess of concrete, steel and other debris. “If we know how plutonium distributes itself, based on our experiments using cerium, we can estimate how much plutonium there is in each piece of debris we cut out of the reactor. This gives us the information we need to safely sort the debris into containers, without having another nuclear accident on our hands,” says Claire.
The Japanese government wants to begin decommissioning the Fukushima reactor by 2022, and the reactor at Chernobyl has waited 33 years to be decommissioned. But the applications of Claire’s work don’t stop there. “The things we’re learning on this project are also useful for decommissioning ‘normal’ reactors around the world.
The UK, France and Germany for example are switching a lot of theirs off, and even though they don’t contain melted down nuclear fuel, they still need to be cleaned up,” says Claire. Doing this as efficiently as possible is highly desirable. For example, the decommissioning budget in the UK is £3bn per year, paid for largely by taxpayers.
Sheffield is the unlikeliest of places to feature a reconstruction of a nuclear meltdown. However, thanks to the work of Claire and her team the scars left by these nuclear disasters might soon fade and we can move into a world where nuclear power is seen to be a safe and reliable source of energy.
By Alicia Shephard, Research Marketing and Content Coordinator
Further information
Dr Claire Corkhill, The University of Sheffield
Research publications
Synthesis, characterisation and corrosion behaviour of simulant Chernobyl nuclear meltdown materials
Media contributions
Engineers develop materials that could help clean-up Chernobyl and Fukushima
Chernobyl's horrifying realism merits its place as TV's top show, Wired
The Truth Behind the Hit TV Series Chernobyl, This Morning
Meet the Sheffield scientist clearing up the world’s nuclear waste, The Yorkshire Post
Funders
European Commission Horizon 2020
University of Sheffield Global Challenges Research Fund
International Atomic Energy Agency
For further information contact:
Sean Barton
Media Relations Officer
University of Sheffield
0114 222 9852
s.barton@sheffield.ac.uk