Dr Andrew Lin
School of Biosciences
Senior Lecturer, School Director of One University
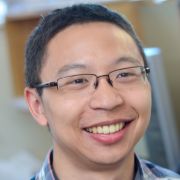
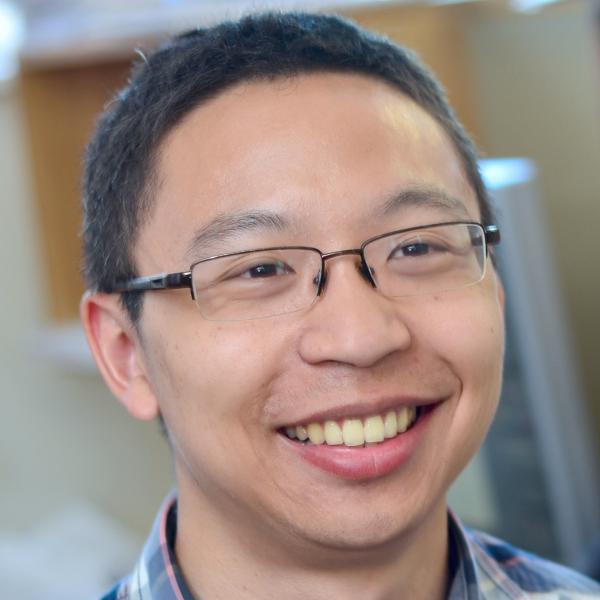
+44 114 222 3643
Full contact details
School of Biosciences
B2 222a
Alfred Denny Building
Western Bank
Sheffield
S10 2TN
- Profile
-
Brief career history:
- 2023 - present: Senior Lecturer, University of Sheffield
- 2019 - 2022: Lecturer, University of Sheffield
- 2015 - 2019: Vice-Chancellor’s Fellow, University of Sheffield
- 2009 - 2015: Postdoctoral fellow, University of Oxford
- 2004 - 2009: PhD, University of Cambridge
- 2000 - 2004: AB Biology, Harvard University
- Research interests
-
We study how the brain represents sensory information to allow it to store unique memories, using the olfactory system of the fruit fly Drosophila melanogaster as a model system.
Olfactory sensory coding and memory
How does the brain recognise sensory stimuli? How does it form distinct memories for different stimuli, even very similar ones? And how does it wire itself up to process information in the best way to achieve these remarkable feats? Our research addresses these fundamental questions using the olfactory system of the fruit fly Drosophila melanogaster. Flies have a much simpler nervous system than humans but are still capable of complex behaviours such as associative memory. This simplicity, combined with the power of fly genetics, makes Drosophila an excellent model system for tackling basic questions about neural circuit function.
Flies can form distinct associative memories for different odours, even very similar ones, and this stimulus-specificity depends on ‘sparse coding’, in which Kenyon cells, the neurons that encode olfactory associative memories, respond sparsely to odours, i.e. only a few neurons in the population respond to each odour. This sparse coding in turn depends on a delicate balance of excitation and inhibition onto Kenyon cells. We are studying how this balance is created and maintained. By improving our understanding of how the brain balances excitation and inhibition, this work may shed light on neurological disorders, like epilepsy, where this balance goes wrong.
Some methods we use:
- In vivo two-photon imaging
- Patch-clamp electrophysiology
- Individual-fly behavioural experiments
- Genetic manipulation of identified neurons
- Transcriptional profiling
- Computational modelling
- Publications
-
Show: Featured publications All publications
Featured publications
Journal articles
- Sensory encoding and memory in the mushroom body: signals, noise, and variability. Learning & Memory, 31(5). View this article in WRRO
- SpaRCe: Improved Learning of Reservoir Computing Systems Through Sparse Representations.. IEEE Trans Neural Netw Learn Syst, 34(2), 824-838.
- Compensatory variability in network parameters enhances memory performance in the Drosophila mushroom body. Proceedings of the National Academy of Sciences, 118(49).
- Multiple network properties overcome random connectivity to enable stereotypic sensory responses. Nature Communications, 11(1), ---.
- Localized inhibition in the Drosophila mushroom body. eLife, 9, ---.
- Mechanisms underlying homeostatic plasticity in the Drosophila mushroom body in vivo. Proceedings of the National Academy of Sciences, 117(28), 16606-16615.
- Inhibitory muscarinic acetylcholine receptors enhance aversive olfactory learning in adult Drosophila. eLife, 8.
All publications
Journal articles
- Mating proximity blinds threat perception. Nature, 634(8034), 635-643. View this article in WRRO
- Sensory encoding and memory in the mushroom body: signals, noise, and variability. Learning & Memory, 31(5). View this article in WRRO
- Relocating coincidence detection for associative learning.. J Physiol, 602(9), 1877-1878.
- Neuroscience: Hacking development to understand sensory discrimination. Current Biology, 33(15), R822-R825.
- SpaRCe: Improved Learning of Reservoir Computing Systems Through Sparse Representations.. IEEE Trans Neural Netw Learn Syst, 34(2), 824-838.
- Compensatory variability in network parameters enhances memory performance in the Drosophila mushroom body. Proceedings of the National Academy of Sciences, 118(49).
- Multiple network properties overcome random connectivity to enable stereotypic sensory responses. Nature Communications, 11(1), ---.
- Localized inhibition in the Drosophila mushroom body. eLife, 9, ---.
- Mechanisms underlying homeostatic plasticity in the Drosophila mushroom body in vivo. Proceedings of the National Academy of Sciences, 117(28), 16606-16615.
- How nitric oxide helps update memories.. Elife, 9.
- Neuronal mechanisms underlying innate and learned olfactory processing in Drosophila. Current Opinion in Insect Science, 36, 9-17. View this article in WRRO
- Inhibitory muscarinic acetylcholine receptors enhance aversive olfactory conditioning in adult Drosophila. eLife, 8.
- Inhibitory muscarinic acetylcholine receptors enhance aversive olfactory learning in adult Drosophila. eLife, 8.
- Neural circuitry coordinating male copulation. eLife, 5(-), ---.
- Sparse, decorrelated odor coding in the mushroom body enhances learned odor discrimination. Nature Neuroscience , 17(4), 559-568. View this article in WRRO
- Sexually Dimorphic Octopaminergic Neurons Modulate Female Postmating Behaviors in Drosophila. Current Biology, 24(7), 725-730.
- Different Kenyon Cell Populations Drive Learned Approach and Avoidance in Drosophila. NEURON, 79(5), 945-956.
- Odor Discrimination in Drosophila: From Neural Population Codes to Behavior. Neuron, 79(5), 932-944.
- Cytoplasmic polyadenylation and cytoplasmic polyadenylation element-dependent mRNA regulation are involved in Xenopus retinal axon development. Neural Development, 4. View this article in WRRO
- A functional equivalent of endoplasmic reticulum and Golgi in axons for secretion of locally synthesized proteins. Molecular and Cellular Neuroscience, 40(2), 128-142.
- Function and regulation of local axonal translation. Current Opinion in Neurobiology, 18(1), 60-68.
- Outsourcing CREB translation to axons to survive. Nature Cell Biology, 10(2), 115-118.
- Local translation and directional steering in axons. The EMBO Journal, 26(16), 3729-3736.
- Asymmetrical β-actin mRNA translation in growth cones mediates attractive turning to netrin-1. Nature Neuroscience, 9(10), 1247-1256.
- Diffraction Pattern Analysis of Bright TRACE Flares. Solar Physics, 198(2), 385-398.
Chapters
- Topographic Maps: Molecular Mechanisms, Encyclopedia of Neuroscience (pp. 1019-1027). Elsevier
- Topographic Maps: Molecular Mechanisms, Encyclopedia of Neuroscience: Volumes 1-11 (pp. V10-1019-V10-1027).
Preprints
- Mating proximity blinds threat perception., Cold Spring Harbor Laboratory.
- Compensatory variability in network parameters enhances memory performance in theDrosophilamushroom body, Cold Spring Harbor Laboratory.
- Exploiting Multiple Timescales in Hierarchical Echo State Networks, arXiv.
- Localized inhibition in theDrosophilamushroom body, Cold Spring Harbor Laboratory.
- SpaRCe: Improved Learning of Reservoir Computing Systems through Sparse Representations, arXiv.
- Learning sparsity in reservoir computing through a novel bio-inspired algorithm, arXiv.
- Sensory encoding and memory in the mushroom body: signals, noise, and variability. Learning & Memory, 31(5). View this article in WRRO
- Grants
-
- European Research Council
- BBSRC
- Wellcome Trust
- Teaching activities
-
- BMS11004 Introduction to Neuroscience
- BMS248 Neural Circuits, Behaviour and Memory
- Level 3 Practical and Dissertation Modules
- Professional activities and memberships
-
- Fellow of the Higher Education Academy (FHEA)
- FENS-Kavli Network of Excellence
- BBSRC Pool of Experts
- Opportunities
We advertise PhD opportunities (Funded or Self-Funded) on FindAPhD.com
For further information and details of other projects on offer, please see the department PhD Opportunities page.