Professor Matt Johnson
School of Biosciences
Professor of Biochemistry, School Director of Research
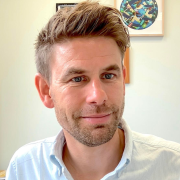
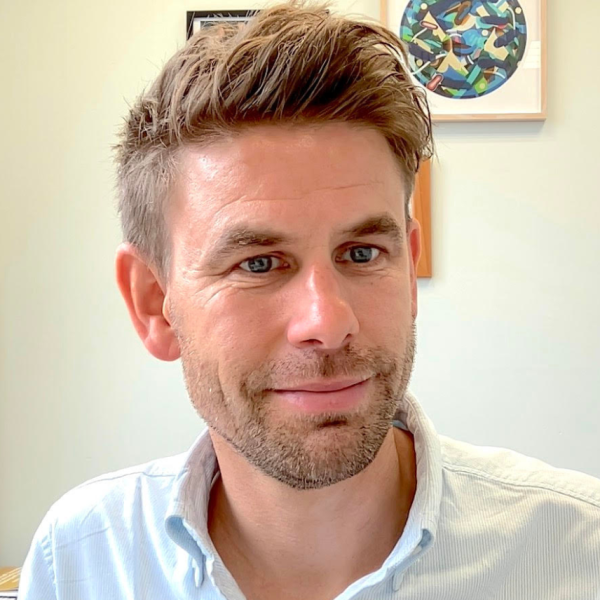
- Profile
-
- 2022 - 2023: Director of Research, School of Biosciences, The University of Sheffield
- 2021 - present: Chair in Biochemistry, School of Biosciences, The University of Sheffield
- 2018 - 2021: Reader in Biochemistry, School of Biosciences, The University of Sheffield
- 2015 - 2017: Lecturer, Dept. of Molecular Biology and Biotechnology, The University of Sheffield
- 2012 - 2017: Krebs Institute Research Fellow, Krebs Institute, University of Sheffield.
- 2012 - 2015: Leverhulme Research Fellow, Dept. of Molecular Biology and Biotechnology, The University of Sheffield
- 2011 - 2012: Project Sunshine Research Fellow, Dept. of Molecular Biology and Biotechnology, The University of Sheffield.
- 2007 - 2011: Postdoctoral Research Associate, Queen Mary University of London.
- 2003 - 2007: PhD ‘The role of the xanthophyll cycle in photoproection in Arabidopsis thaliana’, Dept. of Molecular Biology and Biotechnology, The University of Sheffield.
- Research interests
-
The Johnson Lab is focused on photosynthesis the process that uses solar energy to transform water and carbon dioxide into the energy we consume and the oxygen we breathe. Research within my group falls into three overlapping areas:
Thylakoid membrane structure and dynamics
The chloroplast thylakoid membrane is the site for the initial steps of photosynthesis that convert solar energy into chemical energy, ultimately powering almost all life on earth.
The heterogeneous distribution of protein complexes within the membrane gives rise to an intricate three-dimensional structure that is nonetheless extremely dynamic on a timescale of seconds to minutes.
These dynamics form the basis for the regulation of photosynthesis, and therefore the adaptability of plants to different environments.
We use a multi-faceted approach that includes atomic force, electron and fluorescence microscopies in combination with biochemistry and spectroscopy to probe these organizational details and understand their functional relevance.
Single molecule electron transfer
Small diffusible redox proteins play a ubiquitous role in facilitating electron transfer (ET) in respiration and photosynthesis by shuttling electrons between membrane bound complexes in a redox-dependent manner.
The association of such small redox carrier proteins with their larger membrane-bound partners must be highly specific, yet also readily reversible in order to sustain rapid ET and turnover on microsecond/millisecond timescales.
New developments in force spectroscopy provide the first opportunity to quantify the dynamic forces that sustain these transient interactions and to understand their temporal evolution leading to dissociation.
We have adapted a new atomic force microscopy (AFM) technique PeakForce-QNM (PF-QNM), and have used it to directly monitor these intermolecular interactions at the single molecule level, with sub-millisecond time resolution, and with picoNewton force resolution.
Photoprotective energy dissipation
In photosynthesis, light-harvesting complexes (LHCs) capture solar energy and feed it to the downstream molecular machinery. However, when light absorption exceeds the capacity for utilization, the excess energy can cause damage. Thus, LHCs have evolved a feedback loop that triggers photoprotective energy dissipation.
The critical importance of photoprotection for plant fitness has been demonstrated, as well as its impact on crop yields. However, the mechanisms of photoprotection ― from fast chemical reactions of molecules to slow conformational changes of proteins ― have not yet been resolved.
We are working with partners at MIT and at Okazaki in Japan to study the protein and pigment dynamics that bring about the switch between the photoprotective and light harvesting states.
- Publications
-
Show: Featured publications All publications
Featured publications
Journal articles
- Cytochrome b6f – orchestrator of photosynthetic electron transfer. Biochimica et Biophysica Acta (BBA) - Bioenergetics. View this article in WRRO
- Dynamic thylakoid stacking and state transitions work synergistically to avoid acceptor-side limitation of photosystem I. Nature Plants. View this article in WRRO
- Developmental acclimation of the thylakoid proteome to light intensity in Arabidopsis. The Plant Journal. View this article in WRRO
- Cryo-EM structure of the spinach cytochrome b6 f complex at 3.6 Å resolution. Nature, 575(-), 535-539. View this article in WRRO
- Dynamic thylakoid stacking is regulated by LHCII phosphorylation but not its interaction with PSI. Plant Physiology, 180(4), 2152-2166. View this article in WRRO
- Single-molecule study of redox control involved in establishing the spinach plastocyanin-cytochrome b6f electron transfer complex. Biochimica et Biophysica Acta (BBA) - Bioenergetics, 1860(7), 591-599. View this article in WRRO
- Arabidopsis plants lacking PsbS protein possess photoprotective energy dissipation.. Plant J, 61(2), 283-289.
- Dynamics of higher plant photosystem cross-section associated with state transitions. Photosynthesis Research, 99(3), 173-183.
All publications
Journal articles
- Energetic driving force for LHCII clustering in plant membranes. Science Advances, 9(51).
- Twisted carotenoids do not support efficient intramolecular singlet fission in the orange carotenoid protein. The Journal of Physical Chemistry Letters, 14(26), 6135-6142.
- Cryo-EM structure of the four-subunit
Rhodobacter sphaeroides
cytochrome
bc
1
complex in styrene maleic acid nanodiscs. Proceedings of the National Academy of Sciences, 120(12).
- Zeta-carotene isomerase (Z-ISO) Is required for light-independent carotenoid biosynthesis in the cyanobacterium synechocystis sp. PCC 6803. Microorganisms, 10(9). View this article in WRRO
- Cryo-EM structures of the Synechocystis sp. PCC 6803 cytochrome b6f complex with and without the regulatory PetP subunit. Biochemical Journal. View this article in WRRO
- Changes in supramolecular organization of cyanobacterial thylakoid membrane complexes in response to far-red light photoacclimation.. Sci Adv, 8(6), eabj4437.
- FRET measurement of cytochrome bc1 and reaction centre complex proximity in live Rhodobacter sphaeroides cells. Biochimica et Biophysica Acta (BBA) - Bioenergetics, 1863(2), 148508-148508.
- Inhibition of Arabidopsis stomatal development by plastoquinone oxidation. Current Biology.
- Comparative proteomics of thylakoids from
Arabidopsis
grown in laboratory and field conditions. Plant Direct, 5(10).
- Membrane-dependent heterogeneity of LHCII characterized using single-molecule spectroscopy. Biophysical Journal.
- Genes linking copper trafficking and homeostasis to the biogenesis and activity of the cbb3-type cytochrome c oxidase in the enteric pathogen Campylobacter jejuni. Frontiers in Microbiology, 12. View this article in WRRO
- Cytochrome b6f – orchestrator of photosynthetic electron transfer. Biochimica et Biophysica Acta (BBA) - Bioenergetics. View this article in WRRO
- Dynamic thylakoid stacking and state transitions work synergistically to avoid acceptor-side limitation of photosystem I. Nature Plants. View this article in WRRO
- Developmental acclimation of the thylakoid proteome to light intensity in Arabidopsis. The Plant Journal. View this article in WRRO
- Xanthophyll carotenoids stabilise the association of cyanobacterial chlorophyll synthase with the LHC-like protein HliD. Biochemical Journal. View this article in WRRO
- Just the essentials: photoprotective energy dissipation pared-down. Journal of Experimental Botany, 71(12), 3380-3382.
- Modeling the Role of LHCII-LHCII, PSII-LHCII, and PSI-LHCII Interactions in State Transitions. Biophysical Journal, 119(2), 287-299.
- The relevance of dynamic thylakoid organisation to photosynthetic regulation. Biochimica et Biophysica Acta (BBA) - Bioenergetics, 1861(4), 148039-148039.
- Cryo-EM structure of the spinach cytochrome b6 f complex at 3.6 Å resolution. Nature, 575(-), 535-539. View this article in WRRO
- Dissecting the cytochrome c(2)–reaction centre interaction in bacterial photosynthesis using single molecule force spectroscopy. Biochemical Journal, 476(15), 2173-2190. View this article in WRRO
- Membrane organization of photosystem I complexes in the most abundant phototroph on Earth. Nature Plants, 5(8), 879-889. View this article in WRRO
- Dynamic thylakoid stacking is regulated by LHCII phosphorylation but not its interaction with PSI. Plant Physiology, 180(4), 2152-2166. View this article in WRRO
- Single-molecule study of redox control involved in establishing the spinach plastocyanin-cytochrome b6f electron transfer complex. Biochimica et Biophysica Acta (BBA) - Bioenergetics, 1860(7), 591-599. View this article in WRRO
- Turning the Challenge of Quantum Biology On its Head Biological Control of Quantum Optical Systems. Faraday Discussions, 216, 57-71. View this article in WRRO
- Correlated fluorescence quenching and topographic mapping of Light-Harvesting Complex II within surface-assembled aggregates and lipid bilayers. Biochimica et Biophysica Acta (BBA) - Bioenergetics, 1859(10), 1075-1085. View this article in WRRO
- Author Correction: Dynamic thylakoid stacking regulates the balance between linear and cyclic photosynthetic electron transfer. Nature Plants, 4(6), 391-391. View this article in WRRO
- Metabolic regulation of photosynthetic membrane structure tunes electron transfer function. Biochemical Journal, 475(7), 1225-1233. View this article in WRRO
- Effect of ammonium and high light intensity on the accumulation of lipids in Nannochloropsis oceanica (CCAP 849/10) and Phaeodactylum tricornutum (CCAP 1055/1). Biotechnology for Biofuels, 11(1), 60-60. View this article in WRRO
- Dynamic thylakoid stacking regulates the balance between linear and cyclic photosynthetic electron transfer. Nature Plants, 4, 116-127. View this article in WRRO
- A four state parametric model for the kinetics of the non-photochemical quenching in Photosystem II. Biochimica et Biophysica Acta (BBA) - Bioenergetics, 1858(10), 854-864.
- Cryo-FIB Lift-out Sample Preparation Using a Novel Cryo-gripper Tool. Microscopy and Microanalysis, 23(S1), 844-845.
- Photosynthesis. Essays In Biochemistry, 60(3), 255-273. View this article in WRRO
- Atomic detail visualization of photosynthetic membranes with GPU-accelerated ray tracing. Parallel Computing, 55, 17-27. View this article in WRRO
- An intact light harvesting complex I antenna system is required for complete state transitions in Arabidopsis. Nature Plants, 1(12). View this article in WRRO
- Visualizing the dynamic structure of the plant photosynthetic membrane. Nature Plants, 1(11).
- Interference lithographic nanopatterning of plant and bacterial light-harvesting complexes on gold substrates. Interface Focus, 5(4). View this article in WRRO
- Nanodomains of Cytochrome b6f and Photosystem II Complexes in Spinach Grana Thylakoid Membranes. The Plant Cell, 26(7), 3051-3061. View this article in WRRO
- Reversible Switching between Nonquenched and Quenched States in Nanoscale Linear Arrays of Plant Light-Harvesting Antenna Complexes. Langmuir, 30(28), 8481-8490.
- Integration of energy and electron transfer reactions in plant thylakoids. Biochimica et Biophysica Acta (BBA) - Bioenergetics, 1837, e122-e122.
- Disentangling the low-energy states of the major light-harvesting complex of plants and their role in photoprotection. Biochimica et Biophysica Acta - Bioenergetics.
- Photosynthesis and the environment. Photosynthesis Research, 119(1-2), 1-2.
- The Specificity of Controlled Protein Disorder in the Photoprotection of Plants. BIOPHYSICAL JOURNAL, 105(4), 1018-1026.
- Changes in the energy transfer pathways within photosystem II antenna induced by xanthophyll cycle activity. Journal of Physical Chemistry B, 117(19), 5841-5847.
- Rethinking the existence of a steady-state Δψ component of the proton motive force across plant thylakoid membranes. Photosynthesis Research, 1-10. View this article in WRRO
- Elevated ΔpH restores rapidly reversible photoprotective energy dissipation in Arabidopsis chloroplasts deficient in lutein and xanthophyll cycle activity. Planta, 235(1), 193-204.
- Controlled disorder in plant light-harvesting complex II explains its photoprotective role. Biophysical Journal, 102(11), 2669-2676.
- Higher plant photosystem II light-harvesting antenna, not the reaction center, determines the excited-state lifetime - Both the maximum and the nonphotochemically quenched. Biophysical Journal, 102(12), 2761-2771.
- Exciton annihilation as a probe of the light-harvesting antenna transition into the photoprotective mode. Chemical Physics, 404, 123-128.
- Natural light harvesting: principles and environmental trends. Energy & Environmental Science, 4(5), 1643-1643.
- Photoprotective energy dissipation involves the reorganization of photosystem II light-harvesting complexes in the grana membranes of spinach chloroplasts. Plant Cell, 23(4), 1468-1479.
- Photoprotection in plants involves a change in lutein 1 binding domain in the major light-harvesting complex of photosystem II. Journal of Biological Chemistry, 286(31), 27247-27254.
- Changes in thylakoid membrane thickness associated with the reorganization of photosystem II light harvesting complexes during photoprotective energy dissipation. Plant Signaling and Behavior, 6(9), 1386-1390.
- Origin of absorption changes associated with photoprotective energy dissipation in the absence of zeaxanthin. Journal of Biological Chemistry, 286(1), 91-98.
- Restoration of rapidly reversible photoprotective energy dissipation in the absence of PsbS protein by enhanced ΔpH. Journal of Biological Chemistry, 286(22), 19973-19981.
- Acclimation- and mutation-induced enhancement of PsbS levels affects the kinetics of non-photochemical quenching in Arabidopsis thaliana. Planta, 233(6), 1253-1264.
- Far-red light-regulated efficient energy transfer from phycobilisomes to photosystem i in the red microalga Galdieria sulphuraria and photosystems-related heterogeneity of phycobilisome population. Biochimica et Biophysica Acta - Bioenergetics, 1807(2), 227-235.
- Visualising the mobility and distribution of chlorophyll-proteins in higher plant thylakoid membranes: effects of photoinhibition and protein phosphorylation. The Plant Journal.
- Visualizing the mobility and distribution of chlorophyll proteins in higher plant thylakoid membranes: Effects of photoinhibition and protein phosphorylation. Plant Journal, 62(6), 948-959.
- Xanthophylls as modulators of membrane protein function. Archives of Biochemistry and Biophysics, 504(1), 78-85.
- A theoretical investigation of the photophysical consequences of major plant light-harvesting complex aggregation within the photosynthetic membrane. Journal of Physical Chemistry B, 114(46), 15244-15253.
- Effect of xanthophyll composition on the chlorophyll excited state lifetime in plant leaves and isolated LHCII. Chemical Physics, 373(1-2), 23-32.
- Arabidopsis plants lacking PsbS protein possess photoprotective energy dissipation.. Plant J, 61(2), 283-289.
- Photoprotective energy dissipation in higher plants involves alteration of the excited state energy of the emitting chlorophyll(s) in the light harvesting antenna II (LHCII). Journal of Biological Chemistry, 284(35), 23592-23601.
- Dynamics of higher plant photosystem cross-section associated with state transitions. Photosynthesis Research, 99(3), 173-183.
- The zeaxanthin-independent and zeaxanthin-dependent qE components of nonphotochemical quenching involve common conformational changes within the photosystem II antenna in Arabidopsis. Plant Physiology, 149(2), 1061-1075.
- The photosystem II light-harvesting protein Lhcb3 affects the macrostructure of photosystem II and the rate of state transitions in Arabidopsis. Plant Cell, 21(10), 3245-3256.
- The xanthophyll cycle pool size controls the kinetics of non-photochemical quenching in Arabidopsis thaliana.. FEBS Lett, 582(2), 262-266.
- Induction of efficient energy dissipation in the isolated light-harvesting complex of photosystem II in the absence of protein aggregation. Journal of Biological Chemistry, 283(43), 29505-29512.
- Photosynthetic acclimation: Does the dynamic structure and macro-organisation of photosystem II in higher plant grana membranes regulate light harvesting states?. FEBS Journal, 275(6), 1069-1079.
- The Lhcb protein and xanthophyll composition of the light harvesting antenna controls the ΔpH-dependency of non-photochemical quenching in Arabidopsis thaliana. FEBS Letters, 582(10), 1477-1482.
- Elevated zeaxanthin bound to oligomeric LHCII enhances the resistance of Arabidopsis to photooxidative stress by a lipid-protective, antioxidant mechanism.. J Biol Chem, 282(31), 22605-22618.
- Structure, regulation and assembly of the photosynthetic electron transport chain. Nature Reviews Molecular Cell Biology.
- Exciton-Diffusion Enhanced Energy Capture in an Integrated Nanoscale Platform. ACS Nano.
- Tethering ferredoxin-NADP+ reductase to photosystem I promotes photosynthetic cyclic electron transfer. The Plant Cell.
- Single-Molecule Detection of the Encounter and Productive Electron Transfer Complexes of a Photosynthetic Reaction Center. Journal of the American Chemical Society.
- De novo design of proteins housing excitonically coupled chlorophyll special pairs. Nature Chemical Biology.
- Photosynthetic control at the cytochrome b6f complex. The Plant Cell.
- PGR5 is required to avoid photosynthetic oscillations during light transitions. Journal of Experimental Botany.
STN7 is not essential for developmental acclimation of Arabidopsis to light intensity. The Plant Journal.- High cyclic electron transfer via the PGR5 pathway in the absence of photosynthetic control. Plant Physiology.
- ΔpH dominates proton motive force in plant photosynthesis in both low and high light. Plant Physiology.
- A recombineering pipeline to clone large and complex genes in Chlamydomonas. The Plant Cell.
- Publisher Correction: Dynamic thylakoid stacking and state transitions work synergistically to avoid acceptor-side limitation of photosystem I. Nature Plants.
Conference proceedings papers
- Light-Harvesting Complex II in Controlled Architectures: Visualizing Changes in Fluorescence Lifetimes. Biophysical Journal, Vol. 112(3) (pp 441a-441a)
- Redesigning Photosynthetic Membranes: Development of Bio-Inspired Photonic Nanomaterials. Biophysical Journal, Vol. 110(3) (pp 19a-19a)
Preprints
- Molecular architecture of thylakoid membranes within intact spinach chloroplasts, eLife Sciences Publications, Ltd.
- Molecular architecture of thylakoid membranes within intact spinach chloroplasts, eLife Sciences Publications, Ltd.
- Molecular architecture of thylakoid membranes within intact spinach chloroplasts, Cold Spring Harbor Laboratory.
- Tethering ferredoxin-NADP+reductase to photosystem I promotes photosynthetic cyclic electron transfer, Cold Spring Harbor Laboratory.
- PGR5 is required to avoid photosynthetic oscillations during light transitions, Cold Spring Harbor Laboratory.
- De novo design of energy transfer proteins housing excitonically coupled chlorophyll special pairs, Research Square Platform LLC.
- Energetic driving force for LHCII clustering in plant membranes, Cold Spring Harbor Laboratory.
- Twisted carotenoids do not support efficient intramolecular singlet fission in the orange carotenoid protein, arXiv.
- Supercharged PGR5-dependent cyclic electron transfer compensates for mis-regulated chloroplast ATP synthase, Cold Spring Harbor Laboratory.
- RAS-inhibiting biologics identify and probe druggable pockets including an SII-α3 allosteric site, Cold Spring Harbor Laboratory.
- A recombineering pipeline to clone large and complex genes in Chlamydomonas, Cold Spring Harbor Laboratory.
- Modelling the role of LHCII-LHCII, PSII-LHCII and PSI-LHCII interactions in state transitions, Cold Spring Harbor Laboratory.
- Cytochrome b6f – orchestrator of photosynthetic electron transfer. Biochimica et Biophysica Acta (BBA) - Bioenergetics. View this article in WRRO
- Research group
- Teaching activities
-
Level 1 modules
- MBB161 Biochemistry
- MBB165 Practical Molecular Bioscience 1
Level 2 modules
- MBB266 Biostructures, Energetics and Synthesis (Module Coordinator)
Level 3 modules
- MBB304 Plant Biotechnology
- Professional activities and memberships
-
- 2020: Wain Medal in Biochemistry
- 2018: Biochemical Society Colworth Medal
- 2017: MBB Teaching Prize
- 2016: Society for Experimental Biology President’s Medal in Plant Science