Professor Walter Marcotti
School of Biosciences
Professor of Sensory Neuroscience
Co-director of the Neuroscience Institute
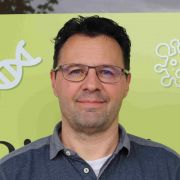
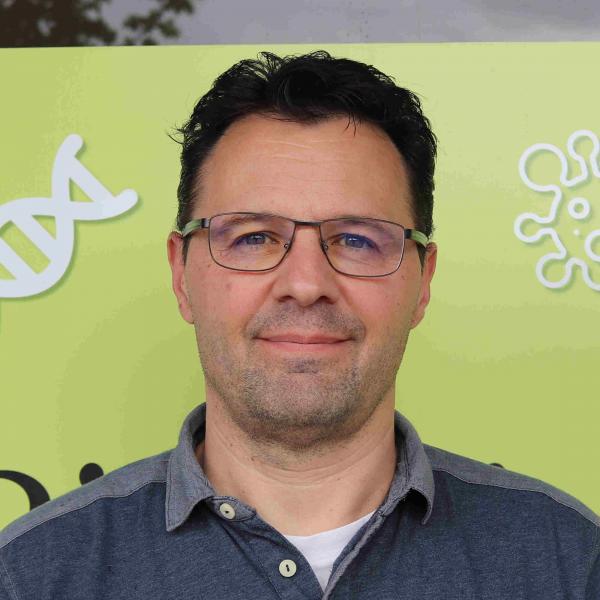
+44 114 222 1098
Full contact details
School of Biosciences
B1 221
Alfred Denny Building
Western Bank
Sheffield
S10 2TN
- Profile
-
- 2012 - present: Professor of Sensory Neuroscience
- 2006 - present: Royal Society University Research Fellow, University of Sheffield, UK.
- 2004 - 2005: Royal Society University Research Fellow, University of Sussex, UK.
- 2001 - 2004: Postdoctoral Fellow, University of Sussex, UK.
- 1997 - 2000: Postdoctoral Fellow, University of Bristol, UK.
- 1994 - 1997: PhD, University of Pavia, Italy.
- 1989 - 1992: University Degree in Biological Science, University of Pavia, Pavia, Italy.
- Research interests
-
- Molecular and physiological mechanisms controlling the functional maturation of the auditory system.
- Mechanoelectrical transduction at the hair cell stereocilia
- Signal processing at ribbon synapses
- Age-related hearing loss
- Mechanisms underlying different forms of hearing loss and deafness
Auditory neuroscience and deafness sensory transduction synaptic transmission
Sensory organs and the neural networks responsible for processing sensory information are supremely well adapted for detecting input from the external environment. Their challenge is to maximize sensitivity and fidelity over a wide dynamic range. The sensory receptors of the mammalian auditory system, the inner hair cells (IHCs), do this with unparalleled temporal precision (kHz range). We know little about the molecular and physiological mechanisms controlling the functional maturation of the auditory system or signal processing at the primary auditory synapses, the IHC ribbon synapses. Crucial to this work, is the need of near-physiological in vitro and the development of in vivo experimental models.
My laboratory is uniquely suited for this task because it is the only one in the world that routinely uses near-physiological conditions for in vitro mammalian cochlear physiology and performs in-vivo electrophysiology from the zebrafish. How biological systems orchestrate their development and how complex signals are processed by mature neuronal networks are major challenges in the quest to understand human biology and disease.
The auditory system provides an ideal model with which to address these questions, primarily because it involves a highly ordered array of a very small number of sensory cells with well-defined neuronal circuitry. It is also a key priority for human health because hearing loss affects more than 360 million people worldwide (WHO 2013), a number that will increase with the aging population.
- Publications
-
Show: Featured publications All publications
Featured publications
Journal articles
- Coordinated calcium signalling in cochlear sensory and non‐sensory cells refines afferent innervation of outer hair cells. The EMBO Journal, 38(9), e99839-e99839.
- The coupling between Ca2+ channels and the exocytotic Ca2+ sensor at hair cell ribbon synapses varies tonotopically along the mature cochlea.. Journal of Neuroscience, 37(9), 2471-2484. View this article in WRRO
- Connexin-Mediated Signaling in Nonsensory Cells Is Crucial for the Development of Sensory Inner Hair Cells in the Mouse Cochlea.. Journal of Neuroscience, 37(2), 258-268. View this article in WRRO
- Connexin-Mediated Signaling in Nonsensory Cells Is Crucial for the Development of Sensory Inner Hair Cells in the Mouse Cochlea. Journal of Neuroscience, 37(2), 258-268.
- In vivo physiological recording from the lateral line of juvenile zebrafish.. Journal of Physiology, 594(19), 5427-5438. View this article in WRRO
- Tmc1 Point Mutation Affects Ca2+ Sensitivity and Block by Dihydrostreptomycin of the Mechanoelectrical Transducer Current of Mouse Outer Hair Cells. Journal of Neuroscience, 36(2), 336-349. View this article in WRRO
- Calcium entry into stereocilia drives adaptation of the mechanoelectrical transducer current of mammalian cochlear hair cells. Proceedings of the National Academy of Sciences, 111(41), 14918-14923.
- Progressive hearing loss and gradual deterioration of sensory hair bundles in the ears of mice lacking the actin-binding protein Eps8L2.. Proc Natl Acad Sci U S A, 110(34), 13898-13903.
All publications
Journal articles
- MYO7A is required for the functional integrity of the mechanoelectrical transduction complex in hair cells of the adult cochlea. Proceedings of the National Academy of Sciences, 122(1).
- Mechanisms driving the functional maturation of the developing mammalian auditory pathway.
- In vivo AAV9-Myo7a gene rescue restores hearing and cholinergic efferent innervation in inner hair cells. JCI Insight, 9(23).
- Pleiotropic brain function of whirlin identified by a novel mutation. iScience, 110170-110170.
- BAI1 localizes AMPA receptors at the cochlear afferent post-synaptic density and is essential for hearing. Cell Reports, 43(4), 114025-114025.
- Correction to ‘Age‐related changes in P2Y receptor signalling in mouse cochlear supporting cells’. The Journal of Physiology, 602(4), 759-759.
- Absence of Embigin accelerates hearing loss and causes sub-viability, brain and heart defects in C57BL/6N mice due to interaction with Cdh23. iScience, 26(10), 108056-108056.
- Recent advances and future challenges in gene therapy for hearing loss. Royal Society Open Science, 10(6).
- AAV-mediated rescue of Eps8 expression in vivo restores hair-cell function in a mouse model of recessive deafness. Molecular Therapy - Methods & Clinical Development, 26, 355-370.
- Signal transmission in mature mammalian vestibular hair cells. Frontiers in Cellular Neuroscience, 16.
- Oncomodulin (OCM) uniquely regulates calcium signaling in neonatal cochlear outer hair cells. Cell Calcium, 105, 102613-102613.
- Grxcr1 regulates hair bundle morphogenesis and is required for normal mechanoelectrical transduction in mouse cochlear hair cells. PLoS ONE, 17(3). View this article in WRRO
- Neuroplastin genetically interacts with Cadherin 23 and the encoded isoform Np55 is sufficient for cochlear hair cell function and hearing. PLoS Genetics, 18(1).
- Current response in CaV1.3–/– mouse vestibular and cochlear hair cells. Frontiers in Neuroscience, 15. View this article in WRRO
- Sensory adaptation at ribbon synapses in the zebrafish lateral line.. J Physiol.
- MET currents and otoacoustic emissions from mice with a detached tectorial membrane indicate the extracellular matrix regulates Ca2+ near stereocilia. The Journal of Physiology.
- Synaptojanin2 mutation causes progressive high-frequency hearing loss in mice. Frontiers in Cellular Neuroscience, 14.
- Exocytosis in mouse vestibular Type II hair cells shows a high‐order Ca2+ dependence that is independent of synaptotagmin‐4. Physiological Reports, 8(14). View this article in WRRO
- Age‐related changes in the biophysical and morphological characteristics of mouse cochlear outer hair cells. The Journal of Physiology. View this article in WRRO
- Generation of otic lineages from integration-free human-induced pluripotent stem cells reprogrammed by mRNAs. Stem Cells International, 2020. View this article in WRRO
- K+ accumulation and clearance in the calyx synaptic cleft of type I mouse vestibular hair cells. Neuroscience, 426, 69-86. View this article in WRRO
- Hair cell maturation is differentially regulated along the tonotopic axis of the mammalian cochlea. The Journal of Physiology, 598(1), 151-170. View this article in WRRO
- Clarin‐2 is essential for hearing by maintaining stereocilia integrity and function. EMBO Molecular Medicine, 11(9). View this article in WRRO
- Gata3 is required for the functional maturation of inner hair cells and their innervation in the mouse cochlea. The Journal of Physiology. View this article in WRRO
- Coordinated calcium signalling in cochlear sensory and non‐sensory cells refines afferent innervation of outer hair cells. The EMBO Journal, 38(9), e99839-e99839.
- Hair Cell Afferent Synapses: Function and Dysfunction. Cold Spring Harbor Perspectives in Medicine, 9(12), a033175-a033175.
- Helios is a key transcriptional regulator of outer hair cell maturation. Nature, 563(7733), 696-700. View this article in WRRO
- Mechanotransduction is required for establishing and maintaining mature inner hair cells and regulating efferent innervation.. Nature Communications, 9. View this article in WRRO
- An allosteric gating model recapitulates the biophysical properties of IK,L expressed in mouse vestibular Type I hair cells.. J Physiol. View this article in WRRO
- Enlargement of ribbons in zebrafish hair cells increases calcium currents, but disrupts afferent spontaneous activity and timing of stimulus onset.. The Journal of Neuroscience, 37(26), 6299-6313. View this article in WRRO
- Corrigendum. The Journal of Physiology, 595(11), 3677-3678.
- Integration of Tmc1/2 into the mechanotransduction complex in zebrafish hair cells is regulated by Transmembrane O-methyltransferase (Tomt).. eLife, 6. View this article in WRRO
- The coupling between Ca2+ channels and the exocytotic Ca2+ sensor at hair cell ribbon synapses varies tonotopically along the mature cochlea.. Journal of Neuroscience, 37(9), 2471-2484. View this article in WRRO
- Connexin-Mediated Signaling in Nonsensory Cells Is Crucial for the Development of Sensory Inner Hair Cells in the Mouse Cochlea.. Journal of Neuroscience, 37(2), 258-268. View this article in WRRO
- Connexin-Mediated Signaling in Nonsensory Cells Is Crucial for the Development of Sensory Inner Hair Cells in the Mouse Cochlea. Journal of Neuroscience, 37(2), 258-268.
- In vivo physiological recording from the lateral line of juvenile zebrafish.. Journal of Physiology, 594(19), 5427-5438. View this article in WRRO
- The acquisition of mechano-electrical transducer current adaptation in auditory hair cells requires myosin VI.. J Physiol, 594(13), 3667-3681. View this article in WRRO
- Distinct roles of Eps8 in the maturation of cochlear and vestibular hair cells. Neuroscience, 328, 80-91.
- Wbp2 is required for normal glutamatergic synapses in the cochlea and is crucial for hearing. EMBO Molecular Medicine, 8(3), 191-207. View this article in WRRO
- Piezo1 haploinsufficiency does not alter mechanotransduction in mouse cochlear outer hair cells. Physiological Reports, 4(3), e12701-e12701. View this article in WRRO
- Tmc1 Point Mutation Affects Ca2+ Sensitivity and Block by Dihydrostreptomycin of the Mechanoelectrical Transducer Current of Mouse Outer Hair Cells. Journal of Neuroscience, 36(2), 336-349. View this article in WRRO
- Absence of Neuroplastin-65 Affects Synaptogenesis in Mouse Inner Hair Cells and Causes Profound Hearing Loss. Journal of Neuroscience, 36(1), 222-234.
- Absence of plastin 1 causes abnormal maintenance of hair cell stereocilia and a moderate form of hearing loss in mice. Human Molecular Genetics, 24(1), 37-49. View this article in WRRO
- Calcium entry into stereocilia drives adaptation of the mechanoelectrical transducer current of mammalian cochlear hair cells. Proceedings of the National Academy of Sciences, 111(41), 14918-14923.
- Transduction without tip links in cochlear hair cells is mediated by ion channels with permeation properties distinct from those of the mechano-electrical transducer channel.. J Neurosci, 34(16), 5505-5514. View this article in WRRO
- In vivo and in vitro biophysical properties of hair cells from the lateral line and inner ear of developing and adult zebrafish.. J Physiol, 592(10), 2041-2058. View this article in WRRO
- A reduction in Ptprq associated with specific features of the deafness phenotype of the miR-96 mutant mouse diminuendo.. Eur J Neurosci, 39(5), 744-756.
- The actin-binding proteins eps8 and gelsolin have complementary roles in regulating the growth and stability of mechanosensory hair bundles of mammalian cochlear outer hair cells.. PLoS One, 9(1), e87331. View this article in WRRO
- Cholinergic efferent synaptic transmission regulates the maturation of auditory hair cell ribbon synapses.. Open Biol, 3(11), 130163. View this article in WRRO
- Progressive hearing loss and gradual deterioration of sensory hair bundles in the ears of mice lacking the actin-binding protein Eps8L2.. Proc Natl Acad Sci U S A, 110(34), 13898-13903.
- Otoferlin couples to clathrin-mediated endocytosis in mature cochlear inner hair cells.. J Neurosci, 33(22), 9508-9519.
- Burst activity and ultrafast activation kinetics of CaV1.3 Ca²⁺ channels support presynaptic activity in adult gerbil hair cell ribbon synapses.. J Physiol, 591(16), 3811-3820. View this article in WRRO
- Presynaptic maturation in auditory hair cells requires a critical period of sensory-independent spiking activity.. Proc Natl Acad Sci U S A, 110(21), 8720-8725.
- Restoration of auditory evoked responses by human ES-cell-derived otic progenitors.. Nature, 490(7419), 278-282. View this article in WRRO
- Development and function of the voltage-gated sodium current in immature mammalian cochlear inner hair cells.. PLoS One, 7(9), e45732. View this article in WRRO
- The resting transducer current drives spontaneous activity in prehearing mammalian cochlear inner hair cells.. J Neurosci, 32(31), 10479-10483.
- Lack of brain-derived neurotrophic factor hampers inner hair cell synapse physiology, but protects against noise-induced hearing loss.. J Neurosci, 32(25), 8545-8553.
- Auditory Function in the Tc1 Mouse Model of Down Syndrome Suggests a Limited Region of Human Chromosome 21 Involved in Otitis Media. PLoS One, 7(2). View this article in WRRO
- Functional assembly of mammalian cochlear hair cells.. Exp Physiol, 97(4), 438-451.
- Prestin-driven cochlear amplification is not limited by the outer hair cell membrane time constant.. Neuron, 70(6), 1143-1154.
- Position-dependent patterning of spontaneous action potentials in immature cochlear inner hair cells.. Nat Neurosci, 14(6), 711-717. View this article in WRRO
- Mutations in protocadherin 15 and cadherin 23 affect tip links and mechanotransduction in mammalian sensory hair cells.. PLoS One, 6(4), e19183. View this article in WRRO
- Eps8 regulates hair bundle length and functional maturation of mammalian auditory hair cells.. PLoS Biol, 9(4), e1001048. View this article in WRRO
- miR-96 regulates the progression of differentiation in mammalian cochlear inner and outer hair cells.. Proc Natl Acad Sci U S A, 108(6), 2355-2360.
- Elementary properties of CaV1.3 Ca(2+) channels expressed in mouse cochlear inner hair cells.. J Physiol, 588(Pt 1), 187-199.
- Synaptotagmin IV determines the linear Ca2+ dependence of vesicle fusion at auditory ribbon synapses.. Nat Neurosci, 13(1), 45-52. View this article in WRRO
- Otoferlin interacts with myosin VI: implications for maintenance of the basolateral synaptic structure of the inner hair cell.. Hum Mol Genet, 18(15), 2779-2790.
- Human fetal auditory stem cells can be expanded in vitro and differentiate into functional auditory neurons and hair cell-like cells.. Stem Cells, 27(5), 1196-1204.
- Functional maturation of the exocytotic machinery at gerbil hair cell ribbon synapses.. J Physiol, 587(Pt 8), 1715-1726.
- TRPML3 mutations cause impaired mechano-electrical transduction and depolarization by an inward-rectifier cation current in auditory hair cells of varitint-waddler mice.. J Physiol, 586(22), 5403-5418.
- Tonotopic variation in the calcium dependence of neurotransmitter release and vesicle pool replenishment at mammalian auditory ribbon synapses.. J Neurosci, 28(30), 7670-7678. View this article in WRRO
- Hair cells-beyond the transducer (vol 209, pg 89, 2006). J MEMBRANE BIOL, 223(3), 173-173.
- Biophysical properties of CaV1.3 calcium channels in gerbil inner hair cells.. J Physiol, 586(4), 1029-1042. View this article in WRRO
- Genetic deletion of SK2 channels in mouse inner hair cells prevents the developmental linearization in the Ca2+ dependence of exocytosis.. J Physiol, 583(Pt 2), 631-646. View this article in WRRO
- Tmc1 is necessary for normal functional maturation and survival of inner and outer hair cells in the mouse cochlea.. J Physiol, 574(Pt 3), 677-698. View this article in WRRO
- Hair cells--beyond the transducer.. J Membr Biol, 209(2-3), 89-118.
- The aminoglycoside antibiotic dihydrostreptomycin rapidly enters mouse outer hair cells through the mechano-electrical transducer channels.. J Physiol, 567(Pt 2), 505-521. View this article in WRRO
- Development and properties of stereociliary link types in hair cells of the mouse cochlea.. J Comp Neurol, 485(1), 75-85.
- Cadherin 23 is a component of the transient lateral links in the developing hair bundles of cochlear sensory cells.. Dev Biol, 280(2), 281-294.
- Increase in efficiency and reduction in Ca2+ dependence of exocytosis during development of mouse inner hair cells.. J Physiol, 563(Pt 1), 177-191. View this article in WRRO
- A transiently expressed SK current sustains and modulates action potential activity in immature mouse inner hair cells.. J Physiol, 560(Pt 3), 691-708. View this article in WRRO
- Effects of intracellular stores and extracellular Ca(2+) on Ca(2+)-activated K(+) currents in mature mouse inner hair cells.. J Physiol, 557(Pt 2), 613-633. View this article in WRRO
- Channel gating forces govern accuracy of mechano-electrical transduction in hair cells.. Proc Natl Acad Sci U S A, 100(26), 15510-15515.
- Sodium and calcium currents shape action potentials in immature mouse inner hair cells.. J Physiol, 552(Pt 3), 743-761. View this article in WRRO
- Developmental changes in the expression of potassium currents of embryonic, neonatal and mature mouse inner hair cells (vol 548, pg 383, 2003). J PHYSIOL-LONDON, 550(3), 996-996.
- Developmental changes in the expression of potassium currents of embryonic, neonatal and mature mouse inner hair cells.. J Physiol, 548(Pt 2), 383-400.
- Beethoven, a mouse model for dominant, progressive hearing loss DFNA36.. Nat Genet, 30(3), 257-258.
- Reduced climbing and increased slipping adaptation in cochlear hair cells of mice with Myo7a mutations.. Nat Neurosci, 5(1), 41-47.
- View this article in WRRO
- Gradients of expression of calcium and potassium currents in frog crista ampullaris.. Pflugers Arch, 442(6), 814-820.
- Transient expression of an inwardly rectifying potassium conductance in developing inner and outer hair cells along the mouse cochlea. Pflügers Archiv - European Journal of Physiology, 439(1), 113-122.
- Transient expression of an inwardly rectifying potassium conductance in developing inner and outer hair cells along the mouse cochlea.. Pflugers Arch, 439(1-2), 113-122.
- Developmental expression of the potassium current IK,n contributes to maturation of mouse outer hair cells.. J Physiol, 520 Pt 3, 653-660.
- View this article in WRRO
- Inactivating and non-activating delayed rectifier K+ currents in hair cells of frog crista ampullaris.. Hear Res, 135(1-2), 113-123.
- Position-dependent expression of inwardly rectifying K+ currents by hair cells of frog semicircular canals.. Neuroreport, 10(3), 601-606.
- Localization of Ca-ATPase in frog crista ampullaris.. Neuroreport, 9(7), 1309-1312.
- Somatotopic nucleocortical projections to the multiple somatosensory cerebellar maps.. Neuroscience, 83(4), 1085-1104.
- Neuropeptide Y (NPY) expression is up-regulated in the rat inferior olive during development.. Neuroreport, 8(17), 3743-3747.
- Neuronal and glial localization of the GABA transporter GAT-1 in the cerebellar cortex.. Neuroreport, 7(18), 2993-2996.
- Inactivation of delayed rectifier K+ current in semicircular canal hair cells.. Neuroreport, 7(13), 2143-2146.
- Potassium currents of pear-shaped hair cells in relation to their location in frog crista ampullaris.. Neuroreport, 7(11), 1841-1845.
- Comparison of three histochemical methods for assaying lactate dehydrogenase in liver.. Acta Histochem, 93(2), 446-452.
- In vivo spontaneous Ca2+ activity in the pre-hearing mammalian cochlea. Nature Communications, 16(1).
- The upregulation of K+ and HCN channels in developing spiral ganglion neurons is mediated by cochlear inner hair cells. The Journal of Physiology.
- Age‐related changes in P2Y receptor signalling in mouse cochlear supporting cells. The Journal of Physiology.
- Oncomodulin regulates spontaneous calcium signalling and maturation of afferent innervation in cochlear outer hair cells. The Journal of Physiology.
- A critical period of prehearing spontaneous Ca
2+
spiking is required for hair‐bundle maintenance in inner hair cells. The EMBO Journal.
- Loss of
Baiap2l2
destabilizes the transducing stereocilia of cochlear hair cells and leads to deafness. The Journal of Physiology.
- Biophysical and morphological changes in inner hair cells and their efferent innervation in the ageing mouse cochlea. The Journal of Physiology.
- Pathophysiological changes in inner hair cell ribbon synapses in the ageing mammalian cochlea. The Journal of Physiology.
- Dynamic coupling of cochlear inner hair cell intrinsic Ca2+action potentials to Ca2+signaling of non-sensory cells.
- Spontaneous and coordinated Ca2+ activity of cochlear sensory and non-sensory cells drives the maturation of OHC afferent innervation.
- TMC2 Modifies Permeation Properties of the Mechanoelectrical Transducer Channel in Early Postnatal Mouse Cochlear Outer Hair Cells. Frontiers in Molecular Neuroscience, 10. View this article in WRRO
- Fine Tuning of CaV1.3 Ca2+ Channel Properties in Adult Inner Hair Cells Positioned in the Most Sensitive Region of the Gerbil Cochlea. PLoS ONE, 9(11), e113750-e113750. View this article in WRRO
- Functional development and regeneration of hair cells in the zebrafish lateral line. The Journal of Physiology.
Chapters
- Physiological recordings from the zebrafish lateral line In Detrich III HW, Westerfield M & Zon LI (Ed.), The Zebrafish: Cellular and Developmental Biology, Part A Cellular Biology (pp. 253-279).
- Functional Development of Hair Cells in the Mammalian Inner Ear, Development of Auditory and Vestibular Systems: Fourth Edition (pp. 155-188).
- Functional Development of Hair Cells in the Mammalian Inner Ear, Development of Auditory and Vestibular Systems (pp. 155-188). Elsevier
- Contributors, Development of Auditory and Vestibular Systems (pp. xi-xiii). Elsevier
- Hair Cells (pp. 1-9). Wiley
- Hair Cells John Wiley & Sons, Ltd
Conference proceedings papers
- SIGNAL PROCESSING BY TRANSDUCER CHANNELS IN MAMMALIAN OUTER HAIR CELLS. Biophysics of the Cochlea
Preprints
- An Open-Source Deep Learning-Based GUI Toolbox For Automated Auditory Brainstem Response Analyses (ABRA), Cold Spring Harbor Laboratory.
- Lack of Oncomodulin Increases ATP-Dependent Calcium Signaling and Susceptibility to Noise in Adult Mice, Cold Spring Harbor Laboratory.
- Oncomodulin Regulates Spontaneous Calcium Signaling and Maturation of Afferent Innervation in Cochlear Outer Hair Cells, Cold Spring Harbor Laboratory.
- Oncomodulin (OCM) uniquely regulates calcium signaling in neonatal cochlear outer hair cells, Cold Spring Harbor Laboratory.
- Neuroplastin genetically interacts with Cadherin 23 and the encoded isoform Np55 is sufficient for cochlear hair cell function and hearing, Cold Spring Harbor Laboratory.
- Coordinated calcium signalling in cochlear sensory and non‐sensory cells refines afferent innervation of outer hair cells. The EMBO Journal, 38(9), e99839-e99839.
- Research group
-
Collaborators
- Prof Steve Brown and Dr Mike Bowl (MRC Harwell, Oxford, UK)
- Prof Corne Kros (University of Sussex, Brighton, UK)
- Grants
- Teaching activities
-
Undergraduate and postgraduate taught modules
Undergraduate:
- BMS335 Sensory Neuroscience (Co-ordinator)
- Level 3 Practical and Dissertation Modules
Masters (MSc):
- BMS6335 Sensory Neuroscience (Co-ordinator)
- Professional activities and memberships
-
- Reviewing editor for Journal of Physiology
- Awards
- Wellcome Trust Investigator (2022)
- Wellcome Trust Senior Investigator (2014)
- Sharpey-Schafer Lecture and Prize (2011) – Physiological Society, Oxford.
- Royal Society University Research Fellowship (2004), The Royal Society, UK.